Page Outline
- More about Monomers
- Biomolecules: Interactive Concept Map
- Monosaccharides
- Carbon Numbering and Directionality
- Monosaccharides to Know: Glucose, Fructose, Deoxyribose, Ribose, G3P
- Monosaccharides Quiz
- Disaccharides
- Disaccharides Quiz
- Polysaccharides
- Carbohydrates: Checking Understanding
Introduction: More about Monomers
In the last tutorial, we met the four biomolecule families: carbohydrates, lipids, proteins, and nucleic acids. Study the table of these macromolecules and their monomers below, and then complete the interactive concept map.
Biomolecule | Monomer |
Carbohydrate | Monosaccharide |
Lipid | Fatty Acid* |
Protein | Amino Acid |
Nucleic Acid | Nucleotide |
*Lipids aren’t polymers in the same way that the other biomolecules are, but thinking of fatty acids as one of the building blocks of lipids is a useful construct for now. We’ll refine it later. |
2. Biomolecules and their Monomers: An Interactive Concept Map
[qwiz qrecord_id=”sciencemusicvideosMeister1961-Biomolecules Concept Map”]
[h]Interactive Concept Map: Biomolecules
[q labels = “top”]
[l]fatty acid
[fx] No. Please try again.
[f*] Correct!
[l]lean meat
[fx] No. Please try again.
[f*] Excellent!
[l]lipids
[fx] No, that’s not correct. Please try again.
[f*] Great!
[l]monosaccharide
[fx] No. Please try again.
[f*] Good!
[l]proteins
[fx] No, that’s not correct. Please try again.
[f*] Great!
[l]nucleic acids
[fx] No. Please try again.
[f*] Correct!
[l]nucleotide
[fx] No. Please try again.
[f*] Great!
[l]starch
[fx] No, that’s not correct. Please try again.
[f*] Correct!
[/qwiz]
Now that we know something about macromolecules in general, let’s focus on carbohydrates.
3. Carbohydrates, part 1: Monosaccharides
Carbohydrates consist of sugars and their polymers, the polysaccharides. You know what sugars are (and I’ll expand on their chemistry below). The polysaccharides include starch, found in bread, pasta, rice, and potatoes; and cellulose, which makes up plant fiber.
Carbohydrates are rich in food energy. Every gram of carbohydrate has about four food calories. You can see this in the food label for Karo pancake syrup, which is made up of sugar (mostly the monosaccharide fructose) and other carbohydrates (mostly soluble starch). Four tablespoons, or 60 mL, provide you with 240 food calories. Since that 60 mL weighs 63 grams, that’s pretty close to the approximation of 4 calories/gram of carbohydrate (63 grams X 4 calories/gram = 252 calories).
To put that in context, you need about 15 food calories to maintain every pound of body weight. That means that if you weighed 150 pounds, you would need about 2250 calories to meet your energy needs. Ten servings of corn syrup (40 tablespoons = 2½ cups, = 0.47 liters) would get you there (though, as we’ll see below, that would not be good for you).
In addition to providing us with food energy, carbohydrates, particularly monosaccharides, show up as parts of other molecules. You’ll see monosaccharides as important pieces of molecules like DNA, the molecule of heredity, or ATP, the moment-to-moment energy currency used in cells.
Above, you learned that the monomers of carbohydrates are monosaccharides, also known as simple sugars. Here are three monosaccharides:
[qwiz style = “width: 650px” qrecord_id=”sciencemusicvideosMeister1961-Biochem: Three Monosaccharides”]
[h]Three Monosaccharides
[q]Take a good look, and identify a few things that these three monosaccharides have in common.
[c]Q2xpY2sgd2hlbiB5b3UmIzgyMTc7cmUgcmVhZHkgdG8gc2VlIHRoZSBhbnN3ZXI=[Qq]
[f]VGhlcmUgYXJlIGEgbG90IG9mIHBvc3NpYmlsaXRpZXMsIGJ1dCBoZXJlIGFyZSBhIGZldyB0byByZW1lbWJlci4=
Cg==- Cg==
- TW9ub3NhY2NoYXJpZGVzIGFyZSBjb21wb3NlZCBvZiB0aHJlZSBlbGVtZW50czogY2FyYm9uLCBoeWRyb2dlbiwgYW5kIG94eWdlbi4= Cg==
- [Qq]Monosaccharides have a general formula of (CH2O)n. What that means is that monosaccharides have a ratio of one carbon atom to two hydrogen atoms to one oxygen atom, multiplied by some multiple. For example, molecule “A” has a formula of C3H6O3, which you could state as (CH2O)3. “B” has a formula of C5H10O5, or (CH2O)5. C” has a formula of C6H12O6 or (CH2O)6.
[/qwiz]
The important point from what’s above is the ratio of hydrogen to oxygen atoms. Complete the sentence below.
[qwiz style=”min-height: 50px !important;” qrecord_id=”sciencemusicvideosMeister1961-Biochem: meaning of ‘carbohydrate'”]
[h]The “Hydrate” in “Carbohydrate”
[q]Think about the word “carbohydrate.” The “carbo” part relates to carbon. The “hydrate” part has something to do with [hangman]
[c]IHdhdGVy[Qq]
[f]IEdvb2Qh[Qq]
[/qwiz]
That’s right: carbohydrate means hydrate of carbon. Add water to carbon, and you have a carbohydrate. That is what happens in photosynthesis: Plant cells take a carbon dioxide molecule and hydrate it, creating glucose and oxygen gas (the oxygen is a waste product). Glucose, whose chemical formula is C6H12O6, is a monosaccharide. Here’s what this looks like as a chemical equation:
6CO2 + 6H2O + light energy → C6H12O6 + 6O2.
You’ll see monosaccharides, and particularly glucose, in many topics in biology, so you should get used to how they’re represented. Here are three representations.
Full structural formula, showing all atoms and all bonds | An abbreviated structural formula.* | Chain form |
![]() |
![]() |
![]() |
* Understanding this representation requires that you envision 1) that each angle vertex has a carbon atom, and 2) that each carbon atom will be saturated with four bonds. So, for example, the carbon atoms at 3 o’clock and 9 o’clock also have hydrogen atoms attached to them (just compare the diagram in the middle with the diagram to the left). |
Note that the chain form is more than a representation. The atoms in a glucose molecule can be arranged as a linear chain, or in the ring configuration. In cells and organisms, the ring configuration is much more commonly found.
4. Carbon numbering and directionality
Biochemists have a system for numbering each of the carbons in a monosaccharide (or any carbon-based molecule). This system lets us talk about molecular structure more precisely, and, it gives us a window into an important concept: directionality.
Start by looking at these structural formulas for the monosaccharide glucose.
Numbered Glucose chain | Numbered Glucose ring |
![]() |
![]() |
Now take a look at this diagram of DNA, the molecule of heredity.
Note the 5′ end in the top left corner, the 3′ end in the top right corner, and so on. If you were talking about DNA, you’d say “5 prime end” or “3 prime end.” These numbers refer to the carbon atoms in the monosaccharide subunits that are part of each DNA monomer. The monomer is called a nucleotide: one part of a nucleotide is the five-carbon sugar deoxyribose, shown in orange above and numbered below.
One reason why this is important is that when cells combine monomers through dehydration synthesis reactions, the enzymes that are involved are usually limited to adding new monomers on one side of the growing molecule. So, for example, when enzymes are copying DNA, they can only add new nucleotides at the 3′ end. That’s what your instructors or textbooks mean when they say that the enzyme moves in a 5′ to 3′ direction.
Additionally, familiarity with this carbon numbering system enables you to understand why molecules have the names they have. For example, when glucose is broken down for energy, the first thing that happens is that an enzyme takes glucose and adds a phosphate group to it. The molecule that results is called glucose-6-phosphate.
[qwiz qrecord_id=”sciencemusicvideosMeister1961-Biochem: Glucose-6-Phosphate”]
[h]Glucose-6-Phosphate
[q]
Here’s what glucose and phosphate look like. Try to put them together and imagine what glucose-6-phosphate would look like. This is a dehydration synthesis reaction, but the phosphate is shown in an ionized form. So, for this exercise, just pull an —OH off of the glucose and connect the glucose to the phosphate.
Glucose | A phosphate group |
[c]Q2xpY2sgdG8gc2VlIHRoZSBhbnN3ZXIu[Qq]
[f]SGVyZSBpdCBpcy4gSWYgeW91IHdlcmUgYWJsZSB0byBpbWFnaW5lIGEgcGhvc3BoYXRlIGdyb3VwIGF0dGFjaGVkIHRvIGNhcmJvbiBhdG9tIG51bWJlciA2LCB0aGVuIHlvdSYjODIxNztyZSBpbiBncmVhdCBzaGFwZQ==[Qq]
[/qwiz]
Here’s another example. During photosynthesis, the monosaccharide that the cell harvests for its matter and energy needs is a three-carbon sugar called glyceraldehyde. When it first appears, it has a phosphate group attached to it, making it glyceraldehyde-3-phosphate, or G3P.
[qwiz qrecord_id=”sciencemusicvideosMeister1961-Biochem: G3P”]
[q]Here’s glyceraldehyde (arranged horizontally) and a phosphate group. Try to put them together and imagine what glyceraldehyde-3-phosphate would look like.
Glyceraldehyde | A phosphate group |
[c]Q2xpY2sgdG8gc2VlIHRoZSBhbnN3ZXI=[Qq]
[f]QWdhaW4sIGFueXRoaW5nIHRoYXQgc2hvd3MgdGhlIHBob3NwaGF0ZSBhdHRhY2hlZCB0byB0aGUgM3JkIGNhcmJvbiBpcyBmaW5lLg==
Cg==[Qq]
[/qwiz]
5. A few monosaccharides to know
Here are a few of the monosaccharides you’ll meet in your study of biology.
We’ll start with glucose and fructose (at right). Both are six-carbon sugars, and structural isomers of one another: they have the same chemical formula (C6H12O6), but their atoms have different arrangements. Both glucose and fructose act as energy sources. They’re fuels. When your body wants to feed your cells, it does so by adding glucose to the bloodstream, either from the food you’ve eaten or from various carbohydrate storage sites within your body. To attract animals to consume their fruits and spread their seeds, plants load their fruits with fructose. Where do they get the fructose? After creating G3P (see above) during photosynthesis, other metabolic pathways lead to the creation of fructose (and glucose).
The idea that too much of a good thing can be a bad thing applies to both glucose and fructose. Your body carefully controls the amount of glucose that circulates in your blood. Inability to control blood glucose can lead to obesity and diabetes. Read this report from the Centers for Disease Control and Prevention if you’re interested in the connection between sugar-sweetened beverages and the risks of being overweight.
Two five-carbon monosaccharides to know are deoxyribose and ribose.
![]() |
![]() |
Deoxyribose | Ribose |
Deoxyribose is the sugar in DNA (deoxyribonucleic acid), the chemical basis of genetic information. To understand its name, take a look at the bottom of the deoxyribose, and compare it to the ribose that’s on the right. Note how the number two carbon in deoxyribose is attached to hydrogen, while the number two carbon in ribose is attached to a hydroxyl group (an –OH). So, take away oxygen from ribose and you have deoxyribose.
Ribose is the sugar in RNA. RNA makes up the genetic material of some viruses, but its main role is to bring genetic information from DNA (in the cell’s chromosomes) to the cytoplasm, where genetic information can be made into protein. Ribose is also the sugar in ATP, the moment-to-moment energy currency of the cell.

A few paragraphs above you met the three-carbon monosaccharide glyceraldehyde. With a phosphate attached to its number 3 carbon, it becomes glyceraldehyde-3-phosphate or G3P. G3P is a key intermediate product in glycolysis, the first step in cellular respiration, which is the process your cells use to convert the chemical bond energy in glucose into ATP. G3P is also the molecule that plant cells make when they convert carbon dioxide into carbohydrates.
![]() |
![]() |
Glyceraldehyde | Glyceraldehyde-3-phosphate (G3P) |
6. Monosaccharides: Checking Understanding
[qwiz use_dataset=”SMV_biochem2_Monosaccharides” dataset_intro=”false” random = “true” qrecord_id=”sciencemusicvideosMeister1961-SMV-monosaccharides quiz”] [h]
Monosaccharides
[i]
[x][restart]
[/qwiz]
7. Carbohydrates, part 2: Disaccharides
Now that we understand the monomers of carbohydrates, we can start exploring combinations of these monomers. The simplest of these are the disaccharides: two connected monosaccharides. All of the disaccharides that you’ll meet below are formed by combining two six-carbon monosaccharides.
[qwiz style=”min-height: 100px !important; border-width: 2px !important;” qrecord_id=”sciencemusicvideosMeister1961-Biochem: Disaccharide Formula”]
[q] If you take two monosaccharides, each with the formula C6H12O6, and combine them through a dehydration synthesis reaction, what will be the chemical formula of the resulting disaccharide?
[c]IFNob3cgbWUgdG hlIGFuc3dlcg==[Qq]
[f]IEM=MTI=SA==MjI=Tw==[Qq]11. Remember that you have to remove a water molecule (with the formula H2O). So, instead of C12H24O12 (a common wrong answer), you have to pull out two hydrogens and one oxygen, leaving you with C12H22O11.
[/qwiz]
Notice that the ratio of hydrogen to oxygen in disaccharides is still 2:1, so we still have a “hydrate of carbon.” However, you should also note that the formula (CH2O)n only applies to monosaccharides. Because of the loss of an H2O during dehydration synthesis reactions, there’s not a 1:2 ratio of carbon to hydrogen.
Disaccharides are generally used for energy transfer. For example, lactose is how sugars get passed from mammalian mothers to their infant offspring. Sucrose is a disaccharide synthesized by plants to transfer energy from the photosynthetic parts of a plant (the leaves) to non-photosynthetic parts (the roots and stem). Many plant saps, including maple syrup, have high concentrations of sucrose.
Maltose
Maltose is made when enzymes combine two glucose monomers (“a” and “c” below). Because this is a dehydration synthesis reaction, a hydrogen atom is removed from one glucose, and a hydroxyl group (–OH) is removed from the second. This is shown by the blue ellipse at “(b).” In addition to producing maltose (“d”), a water molecule (“f”) is also produced. Note that the bond between the two monosaccharides (“e”) is between the number 1 carbon of one glucose and the number 4 carbon of the second. Your instructor or textbook might refer to this bond as an α 1-4 bond (which you would pronounce as “alpha 1-4”). It’s also called a 1-4 glycosidic linkage. The alpha part is important because it relates to the three-dimensional orientation of the two monomers. The key thing to know is that humans (and many other organisms) have hydrolytic enzymes that can break maltose apart into two glucose molecules, which can then be absorbed into our cells and used for energy.
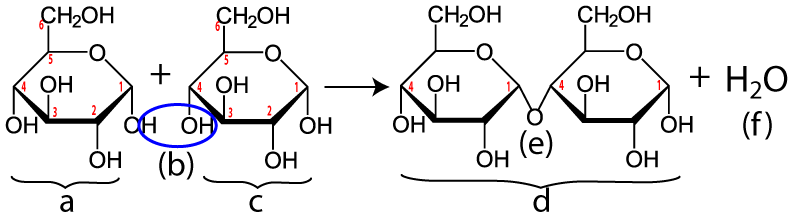
Maltose is found naturally in many foods (beer, sweet potatoes) and it is widely used as a sweetener. But the king of sweeteners is sucrose or table sugar.
Sucrose
|
![]() |
Sucrose is a disaccharide formed by combining glucose (a) and fructose (b). Note that the bond that links these monosaccharides is between the number 1 carbon of glucose and the number 2 carbon of fructose. You’ll see this referred to as an α 1-2 glycosidic linkage, and it’s a bond that our enzymes can easily hydrolyze (break apart), releasing the two monomers which can then be absorbed into our cells.
Sucrose is harvested from sugar cane or sugar beets.
Lactose and Lactose Intolerance
Milk: nutrition facts![]() |
|
Another disaccharide is lactose. Lactose is milk sugar. Notice how in the nutrition facts for a cup of milk, there’s a listing for 12 grams of sugar. That sugar is lactose. Lactose is composed of two linked monosaccharides: galactose (“a” in the diagram above) and glucose (at “b”). The bond linking them together is between the number 1 carbon of galactose and the number 4 carbon in glucose. This bond is a β 1-4 (pronounced “beta 1-4”) glycosidic linkage.
The first food that mammals eat after being born is milk. To digest milk, infant mammals (everything from baby bats to baby whales) produce an enzyme called lactase that breaks the β 1-4 glycosidic linkage, making both glucose and galactose available to the cells of the body.
When mammals are weaned, they stop drinking milk and switch to eating whatever food their species eats. Since it would be wasteful for the body to produce lactose-digesting enzymes when milk is no longer part of an individual’s diet, there’s a natural selection for most mammals to turn off the genes for lactase production after weaning. As a result, most adult mammals are lactose intolerant. Lacking the enzymes to digest lactose, any ingested lactose, instead of being broken down to monosaccharides and absorbed into the body, stays in the gut where it has two unpleasant effects: 1) it draws water into the gut by osmosis, potentially causing diarrhea, and 2) it acts as food for bacteria in the gut, which produce gases (methane gas and hydrogen gas) that cause bloating, cramps, and flatulence (farting). If you’re interested, you can read more about lactose intolerance here.
For almost all adult mammals (horses, lions, giraffes, rats, etc.), lactose intolerance is not a problem. After weaning, these mammals never drink milk. Humans are the exception, with milk from cows, goats, and camels a part of the diet of many cultures. That leads to questions like
- How did lactose tolerance in humans evolve?
- Why is lactose tolerance limited to only some cultures and some individuals within those cultures?
- What’s going on with lactose intolerance in humans?
- Why do we have products like Lactaid milk or Lactaid pills that enable those of us who are lactose intolerant to enjoy milk and milk products?
For answers, we need some evolutionary context. For 90% of our history as a species, we humans were hunter-gatherers. About 10,000 years ago, humans started to develop agriculture, which included, in various parts of the world, the domestication of animals such as cattle, goats, and camels. While the evolutionary origins of how it happened are still being figured out are (read a great article on NPR by clicking here) the result was, in certain human populations, the evolution of lactose tolerance, as milk from domesticated animals became an important part of the diets of these agricultural peoples (and their descendants).
But this process was far from universal. Today, more than half of the human population is lactose intolerant (which, you should remember, is what you would expect from adult mammals). Cultures that are lactose-tolerant are the exception, not the rule, and these cultures are limited to those that originated in Northern Europe, the Middle East, and Eastern Africa. In these ethnic groups, lactase genes stay turned on throughout the lifespan.
But even within these groups, many adults are unable to tolerate lactose (and that includes your author, Mr. W himself). Joining Mr. W are 30 to 50 million U.S. citizens that are also lactose intolerant. To enable these folks to enjoy products like milk and ice cream, companies sell milk that has been treated with lactase, breaking down the lactose. Alternatively, people can take lactase pills when they eat milk products.
8. Disaccharides: Checking Understanding
[qwiz qrecord_id=”sciencemusicvideosMeister1961-SMV-Disaccharides(2)_CFU”]
[h]Disaccharides
[i]
[q] In any disaccharide, the ratio of hydrogen to oxygen is always ____[hangman] to one.
[c]IHR3bw==
Cg==[Qq]
[q] Disaccharides often have the function of energy[hangman]
[c]IHRyYW5zZmVy
Cg==[Qq]
[q] The disaccharide in milk
[hangman]
[c]bGFjdG9zZQ==
Cg==[Qq]
[q] The disaccharide in a sugar cube
[hangman]
[c]c3Vjcm9zZQ==
Cg==[Qq]
[q]Monosaccharides get combined into disaccharides through [hangman] synthesis
[c]ZGVoeWRyYXRpb24=
Cg==[Qq]
[q]Bloating, flatulence, and diarrhea associated with drinking milk are symptoms of possible [hangman] intolerance.
[c]bGFjdG9zZQ==
Cg==[Qq]
[q]Most mammals can only produce lactose-digesting enzymes until they are [hangman].
[c]d2VhbmVk
Cg==[Qq]
[q]Digestion of a disaccharide like maltose results in two [hangman].
[c]bW9ub3NhY2NoYXJpZGVz[Qq]
[/qwiz]
9. Carbohydrates, part 3: Polysaccharides
In the section above, we saw how some enzymes stitch two monosaccharides together to create disaccharides. Other enzymes don’t stop at two but keep on connecting monosaccharides into much longer chains. These chains are called polysaccharides. We’ll examine three: starch, glycogen, and cellulose.
Starch
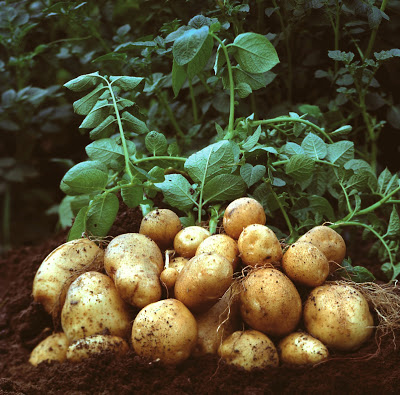
Starch is a polymer of glucose. It’s used by plants for long-term energy storage. Think about a potato. The leaves of plants perform photosynthesis, which creates the three-carbon monosaccharide glyceraldehyde phosphate (G3P). G3P can then be converted into the six-carbon monosaccharide glucose.
Glucose is a high-energy molecule, perfect for powering cellular activity. But because of reasons related to osmosis and water balance, glucose is not well-suited for long-term energy storage. To store chemical energy, cells in the leaves of a potato plant convert glucose into the disaccharide sucrose, which gets transported to an underground part of the stem (called a “tuber”) where the sucrose is converted into starch. This tuber is what we call a potato.
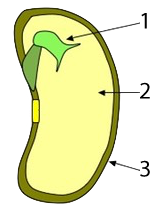
In seeds, such as rice, wheat, and corn, much of the mass of the seed consists of starchy tissue (“2”), the function of which is to sustain the seed’s embryo (“1,” at right) until it sprouts and can start to create its food through photosynthesis (“3” is a protective coat that surrounds and protects the seed).
The simplest form of starch is amylose. It’s a linear chain of glucose molecules.

The chain above has eight glucose monomers. But the chain can go one for hundreds of monomers. To express that, you might see the formulation below. The bracketed glucose monomer with the 300-600 subscript means that you should imagine hundreds of glucose monomers chained together.
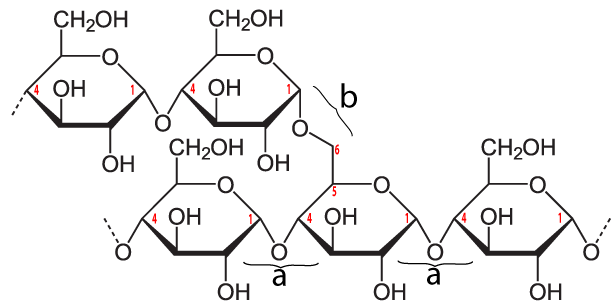
The bonds that hold the glucose monomers together in the amylose form of starch are the same ones that hold the disaccharide maltose together (see above). This bond connects the number 1 carbon of one glucose to the number 4 carbon of the next glucose: an α 1-4 glycosidic linkage. In addition to amylose, there is also a branched form of starch called amylopectin. In amylopectin, one chain of glucose monomers gets linked to another chain by connecting the number 1 carbon of one glucose monomer with the number 6 carbon of another glucose monomer. This bond, called a β 1-6 linkage, is shown at “b” in the diagram to your left. Multiple branches are possible.
The enzymes in our digestive tracts have no difficulty breaking either the α 1-4 bond (at “a”) or the β 1-6 bond (at “b”), meaning that starch can easily be converted into simpler carbohydrates, including glucose. Consequently, while starchy foods evolved to store energy for plants, they are also sources of abundant energy for humans (and many other animals).
You can see evidence of the energy-rich nature of starch to your right, on the nutrition label for a small, 148-gram potato. Most of the potato is water. Of what’s left, notice that the label lists 26 grams of “Total Carbohydrate.” To see how much of that is starch, subtract the two grams of fiber (which we’ll learn about below) and the one gram of sugar. The carbohydrate that remains is starch, and that amounts to 23 grams. Starch, like all digestible carbohydrates, has about 4 calories/gram, so of the 110 total calories, 92 are coming from starch. Eat twenty small potatoes like that, and it’s about all the calories you need. That’s why potatoes were the star of one of my all-time favorite science fiction novels and movies, The Martian, by Andy Weir.
On a more down to Earth level, the energy-rich nature of starch is why tubers like potatoes and sweet potatoes, along with starchy seeds (wheat, corn, and rice), are the staple foods upon which much of modern civilization depends.
Glycogen
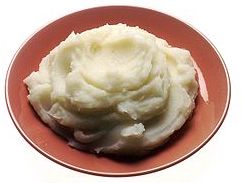
Imagine this scenario. You’re eating a bowl of mashed potatoes. Your digestive system takes the starch, and, in several steps, converts it into glucose by breaking the α 1-4 and β 1-6 bonds that hold the glucose monomers together. After being absorbed into the cells lining your intestines, the glucose diffuses into your bloodstream.
While some of that glucose might be used right away by cells in your muscles, brain, and other organs, your body can also temporarily store that glucose by reconverting it into a polysaccharide. That polysaccharide is called glycogen. It’s stored primarily in your liver and muscles and can constitute a small percentage of an animal’s body weight. Up to a kilogram or two of glycogen can be stored in the liver and muscle tissues of the body of a person my size (I’m about 85 kilograms).
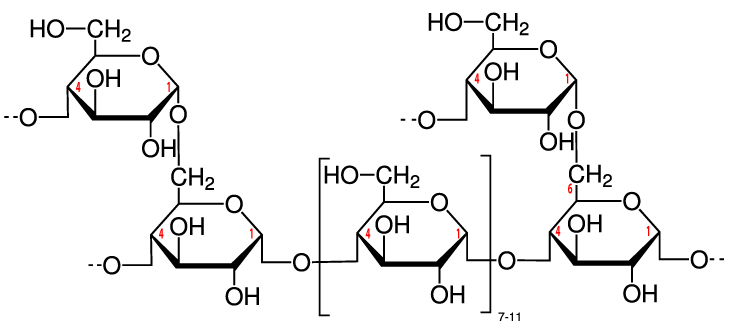
Structurally, glycogen is very similar to amylopectin, the branched form of starch described above. For that reason, glycogen is sometimes called “animal starch.” The only difference is that glycogen tends to be more branched, as you can see in the diagram to your right (imagine each of the glucose monomers towards the top of the diagram as part of longer chains). Like starch, the glucose monomers are held together through α 1-4 and β 1-6 bonds, both of which can be quickly broken down by enzymes in liver and muscle tissue. As a result, when you’re exercising, glycogen is a quick and ready source of energy.
Cellulose
Cellulose is another polysaccharide built from glucose monomers. It’s the most abundant organic polymer on Earth. However, unlike the energy storage polysaccharides starch and glycogen, cellulose’s function is structural. It’s the primary component of plant cell walls, and it’s commonly referred to as “plant fiber.” Cotton is about 90% cellulose. Wood is about 50% cellulose (Wikipedia).
Celery |
|
Celery is a food that’s very high in cellulose. Here’s a nutrition label for one cup of chopped celery. Notice the 1.6 grams of dietary fiber. That’s cellulose.
Notice that celery is a very low-calorie food, with only 14 food calories per cup. By contrast, a cup of corn kernels, which is mostly starch, has over 10 times as many calories (164). If both starch and cellulose are glucose polymers, why do foods with starch have so many more calories/gram than foods with cellulose?
Here’s the structural formula for cellulose.

The link between the glucose monomers is between carbon number 1 of one glucose and carbon number 4 of the next one…which sounds just like the bond that holds together the glucose monomers in starch.
But take another look at the structure of amylose. Notice the difference between amylose and cellulose.

Just to make the differences clear, here are these two polysaccharides, side by side.
The bond between the glucose monomers in cellulose is a β 1-4 linkage, whereas the bond between the glucose monomers in amylose is an α 1-4 linkage. While we have enzymes that can break the α 1-4 bond, we don’t have enzymes that can break down the β 1-4 bond between adjacent glucose monomers. As a result, when you ingest foods high in cellulose, you don’t digest the cellulose into glucose. Consequently, high fiber foods (leaves such as lettuce or spinach), or bran (which is from the hard outer parts of grains) are relatively low in calories. But while fiber doesn’t provide you with calories, it is an important part of the diet. You need fiber to move food through your digestive system (and avoid constipation). Many studies indicate that diets high in fiber protect against various forms of cancer, particularly cancer of the colon (the large intestine). (Johns Hopkins)
Cellulose is not only hard to digest. It’s also strong. Think about materials like cotton fiber, or wood. Part of their strength comes from interactions between cellulose molecules. When arrayed side by side, cellulose strands form hydrogen bonds with one another. In the diagram to the left, these hydrogen bonds are indicated by dashed lines between one hydroxyl group and the next (hydroxyls are the –OH), or between a hydroxyl and the oxygen in a glucose monomer. While hydrogen bonds are weaker than covalent bonds, the collective impact of the bonds between adjacent cellulose strands gives cellulose significant strength.
All vertebrates (animals with backbones) lack the genes that would enable us to produce enzymes to break cellulose down to glucose monomers. However, several mammalian species have formed mutualistic (win-win) partnerships with bacteria that can digest cellulose. These species are called ruminants, a group that includes cows, goats, deer, camels, and sheep. In the insect world, termites have evolved a parallel relationship with a single-celled protist that can also digest cellulose.
![]() |
![]() |
Gazelles and Termites: two examples of organisms that, with the help of mutualistic partners, can digest cellulose (images from Wikipedia) |
10. Carbohydrates: Checking Understanding
Let’s end with a quiz about polysaccharides. However, just to help all of these concepts stick in your memory, I’ll throw in a couple of questions about other carbohydrate topics.
Polysaccharides (and carbohydrates in general)
[i]
[x][restart]
[/qwiz]
Updated, for sync, 8/10/20