Links
- Water Transport in Plants/Transpiration Student Learning Guide
- Transpiration Lab (and viewing leaf stomata)
1. Introduction: it can be a long way up to the top
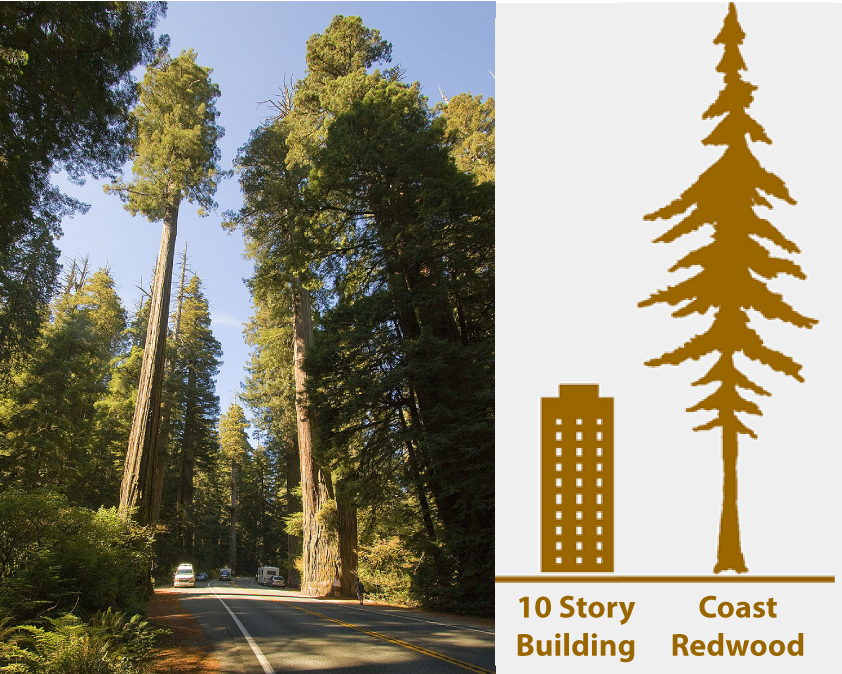
The coast redwood, Sequoia sempervirens, is the world’s tallest tree. These giants live in a narrow strip along the coast of Northern California. The tallest one has been measured at 379.7 feet (115.7 m): that’s as tall as a 30-story building (which is longer than a football field).
Most animals have hearts to pump fluids around their bodies. Plants don’t. So, how can water get from the roots of a plant up to its leaves?
Use the space on your student learning guide to speculate about how this might happen.
2. Plants and Water
Plants, like any living organism, are open systems, constantly exchanging materials with their environments. Plants are particularly open in terms of water, and the amount of water that flows through a tree is astounding. In 1957, Dr. Johnson at Yale University estimated that on a clear 21 °C August day a non-shaded pine tree with an estimated 151 kilograms (333 pounds) of needles lost 181 liters/hour during the 10 hours of maximum sunshine. An oak tree with 39 kg of leaves lost 154 liters/hour during the same period. The water loss peaked from about 2 pm to 5 pm (the hottest part of the day) and dropped off by about 7 pm, with very little water lost during the nighttime. (Adapted from the Naked Scientists); similar data in Principles of Life, Sinauer Associates, page 548).
What are plants using all this water for?
- Photosynthesis: During the light reactions, water is the source of electrons and protons that result in the production of ATP (through chemiosmosis) and NADPH (by reduction of NADP+). During this process, water is consumed (as it’s converted into the waste product oxygen and the hydrogen that’s later used in the Calvin cycle. But this amounts to much less than 1% of the water that flows through plants.
-
C3 plants include most temperate crops (wheat, beans, rice). C4 plants have special adaptations for heat and include maize and sugarcane. CAM plants include cacti, which only perform photosynthesis at night. Evaporative Cooling: The movement of water through plants, as we’ll see, is driven by evaporation. In the same way that the perspiration associated with sweating provides humans with evaporative cooling, evaporation of water in plants has a similar cooling effect. That’s important because, as you can see in the graph to the right, the efficiency of photosynthesis drops when temperatures get too high. That’s because, like almost every other metabolic process, photosynthesis works through enzymes. As the temperature rises beyond an optimum, these enzymes start to denature, causing photosynthesis to slow and eventually cease.
- Maintaining Turgor: Plants maintain their shape and form through osmotic pressure pushing out from their cytoplasm against their cell walls. This outward hydrostatic pressure is called turgor, and it’s an essential part of plant structure. Without sufficient water, plant cells become flaccid, and the plant as a whole goes limp and wilts.
- Transport: Plants, like animals, use their body fluids to transport ions and nutrients from one region of the plant to another. In plants, this transport includes moving sugars from the photosynthetic parts of a plant (the leaves ) to non-photosynthetic parts (like roots, stems, seeds, and fruits). Minerals need to flow from the roots to all other parts of the plant. This flow requires water.
3. Water Potential
The key concept we’ll use to explain water flow in plants is water potential. When you see the term water potential, you should think of water potential energy. That’s because water potential is about water’s tendency to move from one area, where it has higher water potential, to an adjacent area, where the water potential is lower.
Here are two variables related to water potential that we’ll focus on as we look at water movement in plants:
- Osmolarity: adding solute to a solution (increasing its osmolarity) decreases its water potential. You already know about this because of previous work we’ve done learning about how water moves, by osmosis, from hypotonic to hypertonic (use the previous link to review).
- Pressure: Increasing the pressure on a body of water increases water potential. Think about what happens when you wring out a sponge: increased pressure potential in the sponge causes the water to leave the sponge.
Water potential is represented by the Greek letter Ψ, which is pronounced like “sigh.” Ψ is measured in units called megapascals (MPa), and it can be modeled by this equation:
Ψtotal = Ψs + Ψp
water potential = solute potential + pressure potential.
Let’s see this in action. Remember that water always flows from areas of higher potential to areas with lower potential.
[qwiz random=”false” style=”min-height: 350px !important;” qrecord_id=”sciencemusicvideosMeister1961-M32, Water Potential Introduction”] [h]
Water Potential Interactive Reading
[i]
The diagram at left shows a device called a U-tube. Imagine two curved test tubes that can be snapped together to create a U-shaped tube. At the point where the two connect, a selectively permeable membrane has been added that separates the solutions on the right and left sides.
[q multiple_choice=”true”] In the scenario illustrated below, the membrane is permeable to water, but not to the solute (sucrose). If you pour sugar into the left side of U-tube 2, what will happen?
[c]IFdhdGVyIHdpbGwgcmlzZSBvbiB0aGUgcmlnaHQgc2lkZSBvZiB0dWJlIDIgYmVjYXVzZSB0aGUgd2F0ZXIgcG90ZW50aWFsIGlzIGdyZWF0ZXIgb24gdGhlIHNpZGUgd2l0aCBwdXJlIHdhdGVyLg==[Qq]
[f]IE5vLiBBZGRpbmcgc29sdXRlIA==ZGVjcmVhc2VzIHdhdGVyIHBvdGVudGlhbC4gU2luY2Ugd2F0ZXIgYWx3YXlzIGZsb3dzIGZyb20gaGlnaGVyIHdhdGVyIHBvdGVudGlhbCB0byBsb3dlciB3YXRlciBwb3RlbnRpYWwsIHdhdGVyIHdpbGwgZmxvdyBmcm9tIHRoZSByaWdodCBzaWRlIG9mIHR1YmUgMiBpbnRvIGl0cyBsZWZ0IHNpZGUuIENsaWNrICYjODIyMDtOZXh0IFF1ZXN0aW9uJiM4MjIxOyB0byBzZWUgYW4gZXhwbGFuYXRvcnkgZGlhZ3JhbS4=[Qq]
[c]IFdhdGVyIHdpbGwgcmlzZSBvbiB0aGUgbGVmdCBzaWRlIG9mIHR1YmUgMiBiZWNhdXNlIHRoZS B3YXRlciBwb3RlbnRpYWwgaXMgbG93ZXIgb24gdGhlIHNpZGUgd2l0aCBhZGRlZCBzb2x1dGUu[Qq]
[f]IEV4Y2VsbGVudC4gV2F0ZXIgYWx3YXlzIGZsb3dzIGZyb20gaGlnaGVyIHRvIGxvd2VyIHBvdGVudGlhbC4gQWRkaW5nIHN1Y3Jvc2UgZGVjcmVhc2VzIHRoZSB3YXRlciBwb3RlbnRpYWwgb24gdGhlIGxlZnQgc2lkZSwgc28gd2F0ZXIgZmxvd3MgZnJvbSB0aGUgcmlnaHQgc2lkZSAod2l0aCBoaWdoZXIgd2F0ZXIgcG90ZW50aWFsKSB0byB0aGUgbGVmdCBzaWRlICh3aXRoIGxvd2VyIHdhdGVyIHBvdGVudGlhbCkuIENsaWNrICYjODIyMDtOZXh0IFF1ZXN0aW9uJiM4MjIxOyB0byBzZWUgYW4gZXhwbGFuYXRvcnkgZGlhZ3JhbS4=[Qq]
[c]IFRoZSB3YXRlciBsZXZlbCB3aWxsIHJpc2Ugb24gdGhlIGxlZnQgc2lkZSBiZWNhdXNlIHRoZSBhZGRlZCBzdWdhciBpbmNyZWFzZXMgdGhlIHZvbHVtZSBvbiB0aGF0IHNpZGUu[Qq]
[f]IE5vLiBUaGF0JiM4MjE3O3MgdHJ1ZSwgYnV0IGl0JiM4MjE3O3MgYnkgZmFyIG5vdCB0aGUgbW9zdCBpbXBvcnRhbnQgZmFjdG9yLiBUaGUgdGhpbmcgdG8gdGhpbmsgYWJvdXQgaGVyZSBpcyB3YXRlciBwb3RlbnRpYWwuIEFkZGluZyBzb2x1dGUgZGVjcmVhc2VzIHdhdGVyIHBvdGVudGlhbC4gU2luY2Ugd2F0ZXIgYWx3YXlzIGZsb3dzIGZyb20gaGlnaGVyIHdhdGVyIHBvdGVudGlhbCB0byBsb3dlciB3YXRlciBwb3RlbnRpYWwsIHdhdGVyIHdpbGwgZmxvdyBmcm9tIHRoZSByaWdodCBzaWRlIG9mIHR1YmUgMiBpbnRvIGl0cyBsZWZ0IHNpZGUuIENsaWNrICYjODIyMDtOZXh0IFF1ZXN0aW9uJiM4MjIxOyB0byBzZWUgYW4gZXhwbGFuYXRvcnkgZGlhZ3JhbS4=[Qq]
[q] Tube 3 shows what happens if you add solute to the left side of the U-tube. In terms of water potential, what happened is that the water potential on the right side of the tube is now [hangman] than the water potential on the left side of the tube. That’s because adding solute [hangman] water potential.
[c]IGhpZ2hlcg==[Qq]
[f]IEdvb2Qh[Qq]
[c]IGxvd2Vycw==[Qq]
[f]IEV4Y2VsbGVudCE=[Qq]
[q] You should note that while the concept of water potential might be new, the scenario below can be explained just in terms of osmosis. Osmosis is the [hangman] of water. Adding solute made the left side [hangman] to the right side, and water always flows from [hangman] to hypertonic.
[c]IGRpZmZ1c2lvbg==[Qq]
[f]IEdvb2Qh[Qq]
[c]IGh5cGVydG9uaWM=[Qq]
[f]IEdyZWF0IQ==[Qq]
[c]IGh5cG90b25pYw==[Qq]
[f]IEdvb2Qh[Qq]
[q labels = “top”]Quantifying what happens when you add solute to one side of a U-tube is pretty straightforward. The pure water on the right side is not under pressure, and it has no dissolved solutes. Therefore its water potential will be 0 MPa. Start by dragging its water potential next to its Ψ symbol. There’s also no pressure on the solution on the left side, so you can drag the correct number to the Ψp (pressure potential) symbol on the left. Adding solute has lowered the solute potential of the left side, so drag the appropriate number to the Ψs symbol on the left, and then total everything up.
[l]0 MPa
[fx] No, that’s not correct. Please try again.
[f*] Great!
[l]-0.23 MPa
[fx] No, that’s not correct. Please try again.
[f*] Good!
[q]Note that while water potential caused water to flow from the right side to the left, that process won’t continue indefinitely. At a certain point, another force will counteract the force of solution potential: that’s a force that would pull the solution on the left side down. It’s not shown, and we haven’t discussed it, but you can probably guess what it is. It’s a fundamental force that pulls on things, also known as [hangman].
[c]Z3Jhdml0eQ==[Qq]
[q]Pressure is another component of water potential. Imagine that you took a plunger and used it to press down on the left side of the U-tube, as shown in “2.” Predict what will happen, and why.
[c]V2F0ZXIgd2lsbCByaXNlIG9uIHRoZSByaWdodCBzaWRlIG9mIHRoZSB0dWJlICh0aGUgc2lkZSB3aXRob3V0IHRoZSBwbHVuZ2VyKSBiZWNhdXNlIGl0IHdpbGzCoCBoYXZlIGEgaGlnaGVyIHByZXNzdXJlIHBvdGVudGlhbCB0aGFuIHRoZSBzaWRlIHdpdGggdGhlIHBsdW5nZXI=[Qq]
[f]Tm8uIFRoZSBwbHVuZ2VyIGlzIHB1c2hpbmcgZG93biBvbiB0aGUgbGVmdCBzaWRlLiBUaGF0JiM4MjE3O3Mgd2hlcmUgdGhlIHByZXNzdXJlIGlzIGdvaW5nIHRvIGJlLiBUaGF0IHdpbGwgaW5jcmVhc2UgdGhlIHdhdGVyIHBvdGVudGlhbCBvbiB0aGUgbGVmdCBzaWRlLiBJZiB3YXRlciBhbHdheXMgZmxvd3MgZnJvbSBoaWdoZXIgdG8gbG93ZXIgd2F0ZXIgcG90ZW50aWFsLCB0aGVuIGhvdyB3aWxsIHRoZSB3YXRlciBmbG93IGluIHJlc3BvbnNlPyBDbGljayAmIzgyMjA7TmV4dCBRdWVzdGlvbiYjODIyMTsgdG8gc2VlIGFuIGV4cGxhbmF0b3J5IGRpYWdyYW0u[Qq]
[c]VGhlIHdhdGVyIG9uIGJvdGggc2lkZXMgd2lsbCBzdGF5IGF0IHRoZSBzYW1lIGxldmVsIGJlY2F1c2UgdGhlIGZvcmNlIG9mIHRoZSBwbHVuZ2VyIHdpbGwgYmUgb2Zmc2V0IGJ5IHRoZSBmb3JjZSBvZiBncmF2aXR5Lg==[Qq]
[f]Tm8uIEdyYXZpdHkgY2FuIGJlIGEgY29tcG9uZW50IG9mIHdhdGVyIHBvdGVudGlhbC4gQnV0IHlvdSYjODIxNzt2ZSBqdXN0IHNlZW4gaG93IGFkZGluZyBzb2x1dGUgdG8gb25lIHNpZGUgY2F1c2VkIHRoZSBmbHVpZCB0byByaXNlIG9uIHRoYXQgc2lkZSwganVzdCBiZWNhdXNlIG9mIHRoZSBmb3JjZSBvZiBvc21vc2lzLCB3aGljaCBtdXN0IGhhdmUgcHVzaGVkIHdpdGggYSBmb3JjZSB0aGFuIGV4Y2VlZGVkIGdyYXZpdHkmIzgyMTc7cyBkb3dud2FyZCBwdWxsLiBTbywgaWdub3JlIGdyYXZpdHkgZm9yIG5vdy4gSnVzdCB0aGluayB0aGF0IGlmIHRoZSBwbHVuZ2VyIGlzIG9uIHRoZSBsZWZ0IHNpZGUsIHRoZW4gdGhhdCYjODIxNztzIHdoZXJlIHRoZSBwcmVzc3VyZSBpcyBnb2luZyB0byBiZS4gVGhhdCB3aWxsIGluY3JlYXNlIHRoZSB3YXRlciBwb3RlbnRpYWwgb24gdGhlIGxlZnQgc2lkZS4gSWYgd2F0ZXIgYWx3YXlzIGZsb3dzIGZyb20gaGlnaGVyIHRvIGxvd2VyIHdhdGVyIHBvdGVudGlhbCwgdGhlbiBob3cgd2lsbCB0aGUgd2F0ZXIgZmxvdyBpbiByZXNwb25zZT8gQ2xpY2sgJiM4MjIwO05leHQgUXVlc3Rpb24mIzgyMjE7IHRvIHNlZSBhbiBleHBsYW5hdG9yeSBkaWFncmFtLg==[Qq]
[c]VGhlIGRvd253YXJkIGZvcmNlIG9mIHRoZSBwbHVuZ2VyIHdpbGwgaW5jcmVhc2UgdGhlIHdhdGVyIHBvdGVudGlhbCBvbiB0aGUgbGVmdCBzaWRlLiBCZWNhdXNlIHdhdGVyIGFsd2F5cyBmbG 93cyBmcm9tIGhpZ2hlciB0byBsb3dlciB3YXRlciBwb3RlbnRpYWwsIHRoZSB3YXRlciB3aWxsIGZsb3cgZnJvbSBsZWZ0IHRvIHJpZ2h0LCBhbmQgcmlzZSBvbiB0aGUgcmlnaHQgc2lkZS4=[Qq]
[f]TmljZSBqb2IhIFByZXNzdXJlIGluY3JlYXNlcyB3YXRlciBwb3RlbnRpYWwuIFRoYXQgd2lsbCBwdXNoIHRoZSB3YXRlciBhd2F5IGZyb20gdGhlIGxlZnQgc2lkZSBhbmQgcHVzaCBpdCB0b3dhcmQgdGhlIHJpZ2h0IHNpZGUuIENsaWNrICYjODIyMDtOZXh0IFF1ZXN0aW9uJiM4MjIxOyB0byBzZWUgYW4gZXhwbGFuYXRvcnkgZGlhZ3JhbSAoYW5kIHRvIG1vdmUgb24gdG8gdGhlIG5leHQgcXVlc3Rpb24pLg==[Qq]
[q]The downward force of the plunger (pressure) increased the overall [hangman] potential on the left side. Because water always flows from [hangman] to [hangman] water potential, it moves from the left side to the right side.
[c]d2F0ZXI=[Qq]
[c]aGlnaGVy[Qq]
[c]bG93ZXI=[Qq]
[q]Now that we understand the two components of water potential, we can make a few predictions about how this will affect plant cells (which is, after all, why we’re learning this). If you place a flaccid cell in pure water (Ψ = 0.0 MPa, as shown below), what will happen to the cell? Note that in this scenario, the cytoplasm has a Ψs of -1.0 MPa.
[c]VGhlIGNlbGwgd2lsbCBsb3NlIHdhdGVyIGFzIHRoZSB3YXRlciBmbG93cyBmcm9tIHRoZSBjeXRvcGxhc20sIHdoZXJlIHRoZSB3YXRlciBwb3RlbnRpYWwgaXMgbG93ZXIsIHRvIHRoZSBiZWFrZXIsIHdoZXJlIHRoZSB3YXRlciBwb3RlbnRpYWwgaXMgaGlnaGVyLg==[Qq]
[f]Tm8uIExvb2sgYXQgdGhlIHZhbHVlIG9mIM6oIGluIHRoZSBiZWFrZXIuIEl0JiM4MjE3O3MgMC4wIE1QYS4gVGhhdCYjODIxNztzIGhpZ2hlciB0aGFuIHRoZSB2YWx1ZSBvZiDOqCBpbiB0aGUgY2VsbCwgd2hlcmUgaXQmIzgyMTc7cyAtMS4wIE1QYS4gQmVjYXVzZSB3YXRlciBhbHdheXMgZmxvd3MgZnJvbSBoaWdoZXIgdG8gbG93ZXIgd2F0ZXIgcG90ZW50aWFsLCBpdCB3aWxsIGZsb3cgaW50byB0aGUgY2VsbC4=[Qq]
[c]VGhlIGNlbGwgd2lsbCBnYWluIHdhdGVyIGFzIHRoZSB3YXRlciBmbG93cyBmcm9tIHRoZSBiZWFrZXIsIHdoZXJlIHRoZSB3YX RlciBwb3RlbnRpYWwgaXMgaGlnaGVyLCB0byB0aGUgY2VsbCwgd2hlcmUgdGhlIHdhdGVyIHBvdGVudGlhbCBpcyBsb3dlci4=[Qq]
[f]TmljZSBqb2IuIEJlY2F1c2Ugd2F0ZXIgYWx3YXlzIGZsb3dzIGZyb20gaGlnaGVyIHRvIGxvd2VyIHdhdGVyIHBvdGVudGlhbCwgd2F0ZXIgd2lsbCBmbG93IGludG8gdGhlIGNlbGwu[Qq]
[q]Beaker number 2 shows what will happen to beaker number 1 over time. Because the water in the beaker has a water potential of [hangman] MPa, water will flow from the beaker, where the water potential is [hangman] into the cell. What’s lowering the cell’s water potential is the [hangman] in the cytoplasm. In beaker 2, the [hangman] potential, which has risen to 1.0 MPa, is counteracting the -1.0 MPa [hangman] potential, so the cell’s shape has changed. The name for the force of the cytoplasm pushing out against the cell wall is [hangman].
[c]MC4w[Qq]
[c]aGlnaGVy[Qq]
[c]c29sdXRl[Qq]
[c]cHJlc3N1cmU=[Qq]
[c]c29sdXRl[Qq]
[c]dHVyZ29y[Qq]
[x]Now that we understand water potential, let’s look at how water flows through plants.
[/qwiz]
4. Getting water into the root
a. The Big Picture on Water Flow in Plants
The general flow of water into a plant is part of the daily experience of anyone who has ever watered a houseplant or worked on a farm or garden.
[qwiz]
[h]Water flow sequence in plants
[q labels = “top”]Label the sequence of water flow in the illustration of the plant below.
[l]first
[fx] No. Please try again.
[f*] Good!
[l]second
[fx] No. Please try again.
[f*] Excellent!
[l]third
[fx] No. Please try again.
[f*] Correct!
[l]fourth
[fx] No. Please try again.
[f*] Correct!
[l]fifth
[fx] No. Please try again.
[f*] Good!
[q]The first step is to get water to flow from the [hangman] at “b” to the [hangman] at “a.”
[c]c29pbA==[Qq]
[c]cm9vdA==[Qq]
[x]Read below to see how that movement from soil to root occurs.
[/qwiz]
b. Getting into the root
A lot of what you need to know about plant roots you can learn by closely looking at a carrot (which is, of course, a root, as you can see by the inset on the lower left.
Surrounding the carrot, at “e,” is an outer protective layer of cells called an epidermis. Some people like to peel the epidermis off before eating the carrot. Next comes the cortex (at “d”), which is used for the storage of nutrients (such as starch) and water. The cortex ends at the endodermis (at “c”), which is the outer boundary of the vascular cylinder (at “f”). The vascular cylinder consists primarily of two types of conductive tissues (tissues that move water and other solutions). The outermost is called phloem (at “b”), which transports sugars from the leaves to non-photosynthetic parts of the plant (such as the root, the stem, flowers, and fruit). The innermost conductive tissue is the xylem (at “a”), which transports water (with dissolved ions) up from the roots to the rest of the plant.
So how does water enter this system? Here’s a diagram that shows two pathways.
Pathway 1 (in blue) shows water diffusing across the membrane of a root hair (at “a”), an extension of the epidermis (at “b”) that increases the surface area for water absorption. From the epidermis, water will pass from one cell to the next within the root cortex (“c”), following cytoplasmic bridges called plasmodesmata (shown below). After the endodermis (“d”), water will pass into the vascular cylinder, and diffuse into the xylem.
An alternate pathway is shown at “2” (in red). In this pathway, water oozes along the cell walls of the cortex until it reaches the endodermis. At that point, the water encounters a protective waxy barrier that forces the water to diffuse through a cell membrane, and then pass into the xylem. This system ensures that at some point the cell membranes within the root can act as gatekeepers, only allowing material to enter the rest of the plant if it can pass through a selectively permeable cell membrane.
c. Aquaporins facilitate osmosis
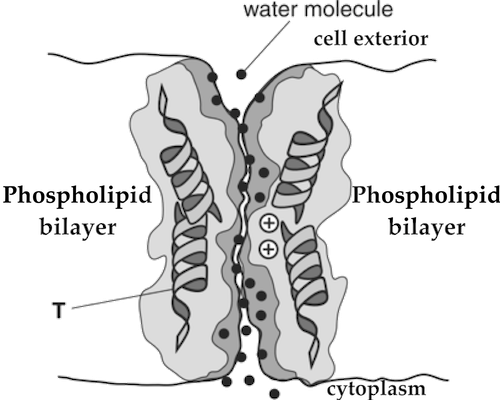
Because water is a polar molecule and the cell membrane has a hydrophobic inner layer, water needs a little help to pass through the root’s cell membranes. Water’s entry into these cells is facilitated by protein channels called aquaporins (“water pores”). Plants can control aquaporin gene transcription and protein abundance in response to environmental stimuli (such as drought), and aquaporin channels can also be opened or closed by a variety of molecular and cellular mechanisms. (See Luu and Maurel, Plant, Cell, and Environment (2005, 28, 85-96).
d. Taking in needed ions against their concentration gradient
Roots also have mechanisms to promote the uptake of needed ions in such a way that these ions can be brought into the plant even when the ionic concentration is greater inside the root than in the soil outside the root. But unlike water flow through aquaporins (which involves facilitated diffusion and has a negative ΔG), moving ions against their concentration gradient takes work. (Note that if you need to review concepts related to cellular work and free energy, you can do so by following this link.)
Plants move ions up their concentration gradients by using ATP-powered proton pumps (“1” at left). These pumps pump protons out of the cytoplasm. This creates a proton gradient across the membrane, and it also creates an electrochemical gradient, with the outside of the membrane having a positive charge relative to the inside. The electrostatic force associated with the electrochemical gradient pulls positively charged ions, such as potassium, through selective channels such as the one shown in “2”. It also creates a situation where cotransport channels can use the kinetic energy of protons diffusing back across the membrane into the cytoplasm to simultaneously move needed negative ions, such as chloride, across the membrane.
5. Checking Understanding: Getting Water into the Root
Before seeing how water makes its ascent to the highest reaches of a plant’s body, let’s consolidate our understanding of what we’ve learned so far.
[qwiz random=”true” qrecord_id=”sciencemusicvideosMeister1961-M32, Getting Water into the Root”]
[h]Quiz: Getting Water into the Root
[i]Biohaiku
Plant Water Transport
No ATP is required
Just solar power
[q] While plants require water for the light reactions, only a very small percentage of the water that flows through plants is consumed during this first phase of [hangman]. Some other functions of the water flowing through plants include 1) cooling that’s caused by water’s [hangman] from the leaves of plants, 2) avoiding wilting by maintaining the outward force in a plant cell called [hangman], and 3) for [hangman] of materials from one part of a plant to another.
[c]IHBob3Rvc3ludGhlc2lz[Qq]
[f]IEdvb2Qh[Qq]
[c]IGV2YXBvcmF0aW9u[Qq]
[f]IENvcnJlY3Qh[Qq]
[c]IHR1cmdvcg==[Qq]
[f]IEdvb2Qh[Qq]
[c]IHRyYW5zcG9ydA==[Qq]
[f]IEdyZWF0IQ==[Qq]
[q] One component of water potential is [hangman] potential, which decreases as the osmolarity of a solution increases.
[c]IHNvbHV0ZQ==[Qq]
[f]IEV4Y2VsbGVudCE=[Qq]
[q] In the diagram below, the vascular cylinder is at
[textentry single_char=”true”]
[c]IG Y=[Qq]
[f]IE5pY2Ugam9iLiBMZXR0ZXIgJiM4MjIwO2YmIzgyMjE7IGlzIHRoZSB2YXNjdWxhciBjeWxpbmRlci4=[Qq]
[c]IEVudGVyIHdvcmQ=[Qq]
[f]IE5vLCB0aGF0JiM4MjE3O3Mgbm90IGNvcnJlY3Qu[Qq]
[c]ICo=[Qq]
[f]IE5vLiBIZXJlJiM4MjE3O3MgYSBoaW50LiBUaGUgdmFzY3VsYXIgY3lsaW5kZXIgY29uc2lzdHMgb2YgdHdvIGNvbmR1Y3RpdmUgdGlzc3VlcyBmb3VuZCBkZWVwIHdpdGhpbiB0aGUgcm9vdDogdGhlIHh5bGVtIGFuZCB0aGUgcGhsb2VtLiBTbywgZmluZCBhIHBhcnQgdGhhdCBjb25zaXN0cyBvZiB0d28gc3VicGFydHMgYW5kIHlvdSYjODIxNztsbCBoYXZlIHRoZSBhbnN3ZXIu[Qq]
[q] In the diagram below, the endodermis is at
[textentry single_char=”true”]
[c]IG M=[Qq]
[f]IEF3ZXNvbWUuIExldHRlciAmIzgyMjA7YyYjODIyMTsgaXMgdGhlIGVuZG9kZXJtaXMsIHdoaWNoIGxpbmVzIHRoZSB2YXNjdWxhciBjeWxpbmRlci4=[Qq]
[c]IEVudGVyIHdvcmQ=[Qq]
[f]IE5vLCB0aGF0JiM4MjE3O3Mgbm90IGNvcnJlY3Qu[Qq]
[c]ICo=[Qq]
[f]IE5vLiBIZXJlJiM4MjE3O3MgYSBoaW50LiBUaGUgZW5kb2Rlcm1pcyBsaW5lcyB0aGUgdmFzY3VsYXIgY3lsaW5kZXIuIFRoZSB2YXNjdWxhciBjeWxpbmRlciBjb25zaXN0cyBvZiB0d28gY29uZHVjdGl2ZSB0aXNzdWVzIGZvdW5kIGRlZXAgd2l0aGluIHRoZSByb290OiB0aGUgeHlsZW0gYW5kIHRoZSBwaGxvZW0uIFNvLCBmaW5kIGEgcGFydCB0aGF0IGNvbnNpc3RzIG9mIHR3byBzdWJwYXJ0cywgYW5kIHRoZW4gZmluZCB0aGUgcGFydCB0aGF0IGxpbmVzIHRoYXQgcGFydCwgYW5kIHlvdSYjODIxNztsbCBoYXZlIGZvdW5kIHRoZSBlbmRvZGVybWlzLg==[Qq]
[q] In the diagram below, the xylem is at
[textentry single_char=”true”]
[c]IG E=[Qq]
[f]IFRlcnJpZmljLiBMZXR0ZXIgJiM4MjIwO2EmIzgyMjE7IGlzIHRoZSB4eWxlbSwgdGhlIHByaW1hcnkgdGlzc3VlIHRoYXQgbW92ZXMgd2F0ZXIgdXAgYSBwbGFudC4=[Qq]
[c]IEVudGVyIHdvcmQ=[Qq]
[f]IE5vLCB0aGF0JiM4MjE3O3Mgbm90IGNvcnJlY3Qu[Qq]
[c]ICo=[Qq]
[f]IE5vLiBIZXJlJiM4MjE3O3MgYSBoaW50LiBUaGUgeHlsZW0gaXMgYSB0aXNzdWUgdGhhdCYjODIxNztzIGZvdW5kIGluIHRoZSBkZWVwZXN0LCBtb3N0IGlubmVybW9zdCBwYXJ0IG9mIHRoZSByb290Lg==[Qq]
[q] In the diagram below, which numbered pathway shows water oozing between cell walls until it arrives at the endodermis?
[textentry single_char=”true”]
[c]ID I=[Qq]
[f]IENvcnJlY3QuIFBhdGh3YXkgJiM4MjIwOzImIzgyMjE7IHNob3dzIHdhdGVyIG9vemluZyBhbG9uZyBjZWxsIHdhbGxzIHVudGlsIGl0IHJlYWNoZXMgdGhlIHNlbGVjdGl2ZWx5IHBlcm1lYWJsZSBlbmRvZGVybWlzLg==[Qq]
[c]IEVudGVyIHdvcmQ=[Qq]
[f]IE5vLg==[Qq]
[c]ICo=[Qq]
[f]IE5vLiBZb3UmIzgyMTc7cmUgbG9va2luZyBmb3IgYSBwYXRod2F5IHdoZXJlIHdhdGVyIG5ldmVyIHBhc3NlcyBpbnRvIGEgY2VsbCB1bnRpbCBpdCByZWFjaGVzIHRoZSBlbmRvZGVybWlzIGF0ICYjODIyMDtkLiYjODIyMTs=[Qq]
[q] In the diagram below, which letter shows an adaptation for increasing root surface area?
[textentry single_char=”true”]
[c]IG E=[Qq]
[f]IFdheSB0byBnby4gTGV0dGVyICYjODIyMDthJiM4MjIxOyBpbmRpY2F0ZXMgYSByb290IGhhaXIsIHRoZSBmdW5jdGlvbiBvZiB3aGljaCBpcyB0byBpbmNyZWFzZSBzdXJmYWNlIGFyZWEu[Qq]
[c]IEVudGVyIHdvcmQ=[Qq]
[f]IE5vLCB0aGF0JiM4MjE3O3Mgbm90IGNvcnJlY3Qu[Qq]
[c]ICo=[Qq]
[f]IE5vLiBZb3UmIzgyMTc7cmUgbG9va2luZyBmb3IgYW4gZXh0ZW5zaW9uIG9mIHRoZSBlcGlkZXJtaXMgKHRoZSBjZWxsIGxheWVyIGF0ICYjODIyMDtiJiM4MjIxOyku[Qq]
[q] In the diagram below, which letter shows the xylem?
[textentry single_char=”true”]
[c]IG Y=[Qq]
[f]IE5pY2UhIExldHRlciAmIzgyMjA7ZiYjODIyMTsgaW5kaWNhdGVzIHRoZSB4eWxlbS4=[Qq]
[c]IEVudGVyIHdvcmQ=[Qq]
[f]IFNvcnJ5LCB0aGF0JiM4MjE3O3Mgbm90IGNvcnJlY3Qu[Qq]
[c]ICo=[Qq]
[f]IE5vLiBZb3UmIzgyMTc7cmUgbG9va2luZyBmb3IgdGhlIGlubmVybW9zdCBsYXllciBvZiBjZWxscy4=[Qq]
[q] Water that’s diffusing into a root has to pass through the cell membranes of the cells of the [hangman] (at “d”) before it can diffuse into the [hangman] (at “f”) and make its way to the rest of the plant.
[c]IGVuZG9kZXJtaXM=[Qq]
[f]IEV4Y2VsbGVudCE=[Qq]
[c]IHh5bGVt[Qq]
[f]IEdvb2Qh[Qq]
[q] [hangman] are protein channels that allow water to [hangman] from the outside of a plant cell into its cytoplasm.
[c]IGFxdWFwb3JpbnM=[Qq]
[f]IEdvb2Qh[Qq]
[c]IGRpZmZ1c2U=[Qq]
[f]IEV4Y2VsbGVudCE=[Qq]
[q] In the diagram below, which number indicates a proton pump?
[textentry single_char=”true”]
[c]ID E=[Qq]
[f]IEV4Y2VsbGVudCEgTnVtYmVyICYjODIyMDsxJiM4MjIxOyBpcyBhIHByb3RvbiBwdW1wLg==[Qq]
[c]IEVudGVyIHdvcmQ=[Qq]
[c]ICo=[Qq]
[f]IE5vLiBIZXJlJiM4MjE3O3MgYSBoaW50LiBQcm90b25zIGNhbiBiZSByZXByZXNlbnRlZCBieSAmIzgyMjA7SA==Kw==LiYjODIyMTs=[Qq]
[q] In the diagram below, which number shows a channel where the ion that’s moving through it is being pulled across by an electrochemical gradient?
[textentry single_char=”true”]
[c]ID I=[Qq]
[f]IE5pY2Ugam9iISBOdW1iZXIgJiM4MjIwOzImIzgyMjE7IHNob3dzIGhvdyBwbGFudHMgY2FuIHVzZSBwcm90b24gcHVtcHMgdG8gdHJhbnNwb3J0IHBvc2l0aXZlIGlvbnMgdXAgYSBjb25jZW50cmF0aW9uIGdyYWRpZW50LiBUaGUgcHVtcGluZyBvZiBwcm90b25zIGNyZWF0ZXMgYW4gZWxlY3Ryb2NoZW1pY2FsIGdyYWRpZW50IHRoYXQgY2FuIHB1bGwgcG9zaXRpdmUgaW9ucyBpbnRvIHRoZSBuZWdhdGl2ZWx5IGNoYXJnZWQgY3l0b3BsYXNtLCBhbmQgdGhhdCYjODIxNztzIGV4YWN0bHkgd2hhdCYjODIxNztzIGhhcHBlbmluZyBpbiBjaGFubmVsIG51bWJlciAmIzgyMjA7Mi4mIzgyMjE7[Qq]
[c]IEVudGVyIHdvcmQ=[Qq]
[c]ICo=[Qq]
[f]IE5vLiBIZXJlJiM4MjE3O3MgYSBoaW50LiBUaG91Z2ggaXQmIzgyMTc7cyBub3QgZGlyZWN0bHkgc2hvd24sIHRoZSBwcm90b24gcHVtcCBhdCAmIzgyMjA7MSYjODIyMTsgY3JlYXRlcyBhbiBlbGVjdHJvY2hlbWljYWwgZ3JhZGllbnQgdGhhdCBjYW4gcHVsbCBwb3NpdGl2ZSBpb25zIGZyb20gb3V0c2lkZSB0aGUgY2VsbCBpbnRvIHRoZSBjZWxsJiM4MjE3O3MgY3l0b3BsYXNtLiBGaW5kIGEgcG9zaXRpdmUgaW9uICh0aGF0JiM4MjE3O3Mgbm90IEg=Kw==KSBtb3ZpbmcgZnJvbSBvdXRzaWRlIHRoZSBjZWxsIHRvIGluc2lkZSwgYW5kIHlvdSYjODIxNztsbCBoYXZlIHRoZSBhbnN3ZXIu[Qq]
[q] In the diagram below, which number shows a cotransport channel?
[textentry single_char=”true”]
[c]ID M=[Qq]
[f]IFRlcnJpZmljISBOdW1iZXIgJiM4MjIwOzMmIzgyMjE7IHNob3dzIGEgY290cmFuc3BvcnQgY2hhbm5lbC4gRmFjaWxpdGF0ZWQgZGlmZnVzaW9uIG9mIHByb3RvbnMgaXMgY291cGxlZCB0byB0aGUgbW92ZW1lbnQgb2YgY2hsb3JpZGUgdXAgaXRzIGNvbmNlbnRyYXRpb24gZ3JhZGllbnQu[Qq]
[c]IEVudGVyIHdvcmQ=[Qq]
[c]ICo=[Qq]
[f]IE5vLiBIZXJlJiM4MjE3O3MgYSBoaW50LiA=Q290cmFuc3BvcnQ=IG1lYW5zIHRoZSB0cmFuc3BvcnQgb2YgdHdvIHRoaW5ncyB0b2dldGhlci4gV2hpY2ggbWVtYnJhbmUgcHJvdGVpbiBpcyB0cmFuc3BvcnRpbmcgdHdvIHRoaW5ncyBhdCB0aGUgc2FtZSB0aW1lPw==[Qq]
[q] In the diagram below, the transport protein that’s directly using active transport is at number
[textentry single_char=”true”]
[c]ID E=[Qq]
[f]IFRlcnJpZmljISBOdW1iZXIgJiM4MjIwOzEmIzgyMjE7IHNob3dzIGEgcHJvdG9uIHB1bXAgdGhhdCYjODIxNztzIGRpcmVjdGx5IHVzaW5nIHRoZSBjb252ZXJzaW9uIG9mIEFUUCB0byBBRFAgYW5kIFA=aQ==LCB0byBwdW1wIHByb3RvbnMgZnJvbSBsb3dlciBjb25jZW50cmF0aW9uIChpbiB0aGUgY3l0b3BsYXNtKSB0byBoaWdoZXIgY29uY2VudHJhdGlvbiAob3V0c2lkZSB0aGUgbWVtYnJhbmUpLg==[Qq]
[c]IEVudGVyIHdvcmQ=[Qq]
[c]ICo=[Qq]
[f]IE5vLiBIZXJlJiM4MjE3O3MgYSBoaW50LiBGaW5kIHRoZSBtZW1icmFuZSBwcm90ZWluIHRoYXQmIzgyMTc7cyBwb3dlcmluZyBpdHMgYWN0aXZpdGllcyBieSBjb252ZXJ0aW5nIEFUUCB0byBBRFAgYW5kIFA=aQ==Lg==[Qq]
[/qwiz]
6. From the Root to the Shoot
In a plant, everything above the root (area 2) is called the shoot (area 1). In a Coast Redwood, that shoot might be 30 stories up in the air. How does the water rise from the root to the shoot?
a. Pushing up from Below?
Let’s consider two hypotheses about pushing water up the stem.
- Pumping from below? Plants have no pumping hearts that can create the pressure that would pump fluids up into the shoot, But in the late 1800s, scientists wondered if there might be some distributed, cellular/molecular pumping system that might push water up the stem.
This hypothesis was eliminated by an experiment by Eduard Strasburger. Strasburger took woody stems from small trees and severed the stem from the roots while leaving the upper part of the stem and the leaves intact. He then immersed the tree’s stem in a concentrated solution of a plant poison such as copper sulfate. The tree sucked up the poisonous solution, which killed the living cells in the stem, branches, and finally the leaves. Strasburger observed that the dead tree, with leaves intact, could continue to suck up water for several weeks, and could even lift solutions of a different color up the dead stem (source: http://6e.plantphys.net/essay04.02.html. See also Principles of Life, p. 548).
-
Capillary action Capillary action? If you take a thin, glass tube that’s open at both ends and place it in water, the water will climb up the walls of the tube. The thinner the tube, the higher the water will climb. Why? It’s because water molecules are polar, and form hydrogen bonds with one another, and with other polar substances. Hydrogen bonds between the water and the inner walls of the xylem tube (adhesion), along with hydrogen bonding between the water molecules themselves (cohesion), can propel the water up the tube.
xylem Much of a plant is composed of just these types of thin-diameter tubes. These tubes make up the xylem, a plant tissue that provides a pathway for the transport of water up a stem.
Here are a few things to know about xylem. First, the tubes that make it up are about 100 micrometers in diameter (that’s a tenth of a millimeter, so just visible to the naked eye). Second, the xylem tubes are open at their ends, so that they form a continuous pathway for water, all the way from the root to the leaves. Part of the reason behind this structure is that the cells that form these tubes are dead at maturity, consisting of nothing more than a cell wall impregnated with lignin, a polymer that’s responsible for giving wood much of its strength. When you’re holding a board of wood, you’re mostly holding xylem.
However, capillary action can only propel water up a tube for a relatively short distance: about 15 centimeters up a stem. If we’re talking about a redwood tree, that’s less than 0.1% of the journey to the top of the shoot.
So, no force is pushing up water from below. What about pulling from above?
b. Transpiration-Cohesion-Tension Theory
For about the last 100 years, upward water flow in plants has been explained through the transpiration-cohesion-tension theory.
Transpiration is the upward movement and evaporation of water from the uppermost structures of a plant, primarily its leaves. As we’ve seen, enormous quantities of water exit a plant’s leaves. So, let’s start there and see how water moves to a plant’s upper reaches. Note that you can scroll the text below the following diagram.
We’ve looked at the structure of leaves in our study of photosynthesis (you can follow this link for a more detailed review). For now, what you need to know is that leaves have a water-impermeable upper epidermis (at “a”) that’s sealed by a waxy cuticle (not shown in this diagram). Water comes into the leaf through the xylem (at “c”). From the xylem, water diffuses to the cells of the leaf.
Unlike the upper epidermis, the lower epidermis (“d”) has openings (“e”) to allow carbon dioxide to enter the leaf for photosynthesis. These openings are called stomata, and they connect to the leaf’s inner airspaces. At the interface between the cells and the airspaces, water molecules will evaporate. The rate of evaporation will be determined by a variety of variables, including temperature, humidity level, and wind.
As we’ll see below, plants can regulate the opening and closing of their stomata. But as long as the stomata are open, water vapor will follow its water potential gradient, and move from its higher potential in the moist innards of the leaf (about -0.2 MPa) to the much drier air outside the stomata, where the water potential is about -58.5 MPa (values from Principles of Life, Sinauer Associates, p. 551).
The water molecules in the leaf are connected by hydrogen bonds. That’s the cohesion part of the transpiration-cohesion-tension theory. As water molecules evaporate from the film of water lining the cells inside the leaf, they create tension in that film of water. Tension is the opposite of pressure. When you pull on a string, you’ve created tension. So as one molecule evaporates from the film of water lining the cells, the water molecule right behind it moves in to take its place.
This water molecule, in turn, pulls on the water molecules behind it. This upward flow is indicated by the letter “j” in the callout of the stem, which is showing a column of water molecules moving up the xylem. In addition to the cohesion that’s connecting these water molecules, there’s also adhesion between the water molecules and the cellulose walls of the xylem.
These forces of tension, cohesion, and adhesion continue down to the start of the xylem in the plant’s roots, shown at letter “i” in the callout of the root (which is also showing a root hair at “f,” a soil particle at “g,” and a water molecule at “h”).
Note that all along the way from the root to the shoot, water is following a water potential gradient. You can see this in the diagram at the left, which you should study for a moment before proceeding.
While the primary components of water potential aren’t separated in this diagram, keep in mind that solute potential is the main force that gets water into the root, but once water is in the root xylem it’s pressure potential that’s pulling the water up, sucking water from the root into the shoot. The key point is that the pressure is negative: it’s not pumping pressure from below, but tension from above, generated by transpiration, that’s pulling water up the stem, into the leaves, and out into the atmosphere.
Got it? Take the quiz below.
7. Checking Understanding: Transpiration Cohesion-Tension
[qwiz random=”true” qrecord_id=”sciencemusicvideosMeister1961-M32_transpiration-cohesion-tension”]
[h]Transpiration-Cohesion-Tension Quiz
[i]
[q] Water in plants flows from the [hangman] (shown at “2”) to the [hangman] (shown at “1”).
[c]IHJvb3Q=[Qq]
[c]IHNob290[Qq]
[q] Water in plants exits the leaves through a process called [hangman]. The pores through which water leaves are called [hangman].
[c]IHRyYW5zcGlyYXRpb24=[Qq]
[c]IHN0b21hdGE=[Qq]
[q] The theory explaining the ascent of water through a plant has a three-part name. The first part is [hangman], which refers to the evaporation of water from the leaves (at “d”). The second part is [hangman], which refers to how water molecules bond with one another, pulling one another up the stem. The third part is [hangman], which refers to the negative pressure (suction) generated by the evaporation of water.
[c]IHRyYW5zcGlyYXRpb24=[Qq]
[c]Y29oZXNpb24=[Qq]
[c]dGVuc2lvbg==[Qq]
[q]The tubes through which water ascends from a plant’s root to its shoot are collectively known as [hangman]. The cells that make up these tubes are [hangman] at maturity, consisting only of cell walls.
[c]eHlsZW0=[Qq]
[c]ZGVhZA==[Qq]
[q]The diagram below demonstrates [hangman] action. The key force that enables water to ascend these tubes is the [hangman] between the water molecules in the column, and the [hangman] between the water molecules and the walls of the tubes.
[c]Y2FwaWxsYXJ5[Qq]
[c]Y29oZXNpb24=[Qq]
[c]YWRoZXNpb24=[Qq]
[q] In the diagram below, the water-impermeable upper epidermis is at
[textentry single_char=”true”]
[c]IG E=[Qq]
[f]IFRlcnJpZmljISBUaGUgbGV0dGVyICYjODIyMDthJiM4MjIxOyBzaG93cyB0aGUgdXBwZXIgZXBpZGVybWlzLCB0aGUgd2F0ZXItaW1wZXJtZWFibGUgdXBwZXIgY2VsbCBsYXllciBpbiBhIGxlYWYu[Qq]
[c]IEVudGVyIHdvcmQ=[Qq]
[c]ICo=[Qq]
[f]IE5vLiBIZXJlJiM4MjE3O3MgYSBoaW50LiBJZiBhIGxlYWYgd2VyZSBhIHNhbmR3aWNoLCB0aGlzIHdvdWxkIGJlIHRoZSBwaWVjZSBvZiBicmVhZCBvbiB0b3Au[Qq]
[q] In the diagram below, the stomata are shown at
[textentry single_char=”true”]
[c]IG U=[Qq]
[f]IE5pY2UgTGV0dGVyICYjODIyMDtlJiM4MjIxOyBzaG93cyB0aGUgc3RvbWF0YS4=[Qq]
[c]IEVudGVyIHdvcmQ=[Qq]
[c]ICo=[Qq]
[f]IE5vLiBIZXJlJiM4MjE3O3MgYSBoaW50LiBUaGUgc3RvbWF0YSBhcmUgb3BlbmluZ3MgaW4gdGhlIGxvd2VyIGVwaWRlcm1pcy4gJiM4MjIwO0VwaWRlcm1pcyYjODIyMTsgbWVhbnMgc2tpbiwgYW5kIHlvdSYjODIxNztyZSBsb29raW5nIGZvciB0aGUgZXBpZGVybWlzIGluIHRoZSBsb3dlciBwYXJ0IG9mIHRoZSBsZWFmLg==[Qq]
[q] In the diagram below, leaf xylem is shown at
[textentry single_char=”true”]
[c]IG M=[Qq]
[f]IE5pY2UgTGV0dGVyICYjODIyMDtjJiM4MjIxOyBzaG93cyB0aGUgbGVhZiB4eWxlbS4=[Qq]
[c]IEVudGVyIHdvcmQ=[Qq]
[c]ICo=[Qq]
[f]IE5vLiBIZXJlJiM4MjE3O3MgYSBoaW50LiBUaGUgeHlsZW0gaXMgYSBjb25kdWN0aW5nIHR1YmUuIFdoYXQgbG9va3MgbGlrZSBhIHR1YmUgaW5zaWRlIHRoZSBsZWFmPw==[Qq]
[q] In the diagram below, water moving through the xylem in the stem is shown at
[textentry single_char=”true”]
[c]IG o=[Qq]
[f]IEV4Y2VsbGVudC7CoCBMZXR0ZXIgJiM4MjIwO2omIzgyMjE7IHNob3dzIHRoZSB4eWxlbSBtb3ZpbmcgdGhyb3VnaCB0aGUgc3RlbS4=[Qq]
[c]IEVudGVyIHdvcmQ=[Qq]
[c]ICo=[Qq]
[f]IE5vLiBIZXJlJiM4MjE3O3MgYSBoaW50LiBJdCYjODIxNztzIGluIHRoZSBzdGVtIChhcyBvcHBvc2VkIHRvIHRoZSBsZWFmIG9yIHJvb3RzKS4=[Qq]
[q] In the diagram below, the lowest water potential would be at
[textentry single_char=”true”]
[c]IG U=[Qq]
[f]IFdheSB0byBnby4gV2F0ZXIgbW92aW5nIHRocm91Z2ggYSBwbGFudCBpcyBmb2xsb3dpbmcgYSB3YXRlciBwb3RlbnRpYWwgZ3JhZGllbnQuIFRoZSBsb3dlc3Qgd2F0ZXIgcG90ZW50aWFsIGlzIGluIHRoZSBvdXRzaWRlIGFpciwgYW5kIGp1c3QgYmVsb3cgdGhhdCB3b3VsZCBiZSB0aGUgd2F0ZXIgcG90ZW50aWFsIGluIHRoZSBhaXIgc3BhY2VzIGJlaGluZCB0aGUgc3RvbWF0YS4=[Qq]
[c]IEVudGVyIHdvcmQ=[Qq]
[c]ICo=[Qq]
[f]IE5vLiBIZXJlJiM4MjE3O3MgYSBoaW50LiBXYXRlciBtb3ZpbmcgdGhyb3VnaCBhIHBsYW50IGlzIGZvbGxvd2luZyBhIHdhdGVyIHBvdGVudGlhbCBncmFkaWVudC4gV2F0ZXIgZmxvd3MgZnJvbSB0aGUgaGlnaGVzdCB3YXRlciBwb3RlbnRpYWwgdG8gdGhlIGxvd2VzdC4gRm9yIHdhdGVyIHRvIGxlYXZlIHRoZSBwbGFudCwgdGhlIGxvd2VzdCB3YXRlciBwb3RlbnRpYWwgd291bGQgaGF2ZSB0byBiZSB3aGVyZT8=[Qq]
[/qwiz]
8. Control of Water Loss
a. Regulation via Stomata
Plants can regulate the rate of transpiration by controlling the opening and closing of their stomata.
The stomata consist of two guard cells (shown at “1”). By pumping out protons (as shown in diagram “A”), guard cells can create an electrochemical gradient that pulls in ions such as potassium and chloride. High levels of these ions lower the water potential inside the guard cells, and water will flow into the guard cells from the surrounding epidermal cells (as shown in “B”). This causes the guard cells to buckle outward, creating the stoma (singular of “stomata”).
This is what happens during the daytime: light induces the proton pumping that creates the osmotic situation just described. This adaptation allows plants to open their stomata so that they can take in carbon dioxide as they photosynthesize.
During the nighttime, photosynthesis ceases. Lack of light shuts down the guard cells’ proton pumps. As a result (shown in image “C”), potassium and chloride diffuse out of the guard cells, and water follows by osmosis, causing the guard cells to change shape, closing the stomata.
In addition to light and dark, several other environmental cues influence whether stomata are open or closed.
- When CO2 levels go down inside the air spaces within a leaf, stomata respond by opening up.
- Drought causes the release of a plant hormone called abscisic acid that signals guard cells to close their stomata.
- Stomata are also controlled by an internal circadian rhythm that will cause them to open and close on a 24-hour timetable, even in the absence of environmental stimuli.
b. Environmental Effects on Transpiration
Along with inducing the opening and closing of stomata, the environment will affect rates of transpiration in plants. For example, a hot, dry day will increase transpiration by lowering the water potential of the outside air. Wind also increases transpiration. On the other hand, increased humidity and lower temperatures will decrease rates of transpiration.
c. Evolutionary Adaptations
A plant’s ability to preserve water is strongly selected by evolution.
Plants such as cacti which have adapted to life in deserts have:
- Increased the amount of waxy cuticle on all exposed parts
- Reduced their leaves to spines. Instead of using leaves, they carry out photosynthesis in their stems.
- Sheltered their stomata in sunken pits that are covered by hairs that reduce airflow.
- Evolved a modified photosynthetic pathway in which they absorb carbon dioxide during the nighttime, enabling them to keep their stomata closed during the daytime.
Other desert plants have modified life cycles in which they can germinate, produce flowers, and reproduce new seeds all within a brief period following rain, with seeds remaining dormant until the next rainstorm.
9. Control of Transpiration (and other related issues): Cumulative Quiz
[qwiz random=”true” qrecord_id=”sciencemusicvideosMeister1961-M32, Control of Transpiration”]
[h]Transpiration/Control of Transpiration Cumulative Quiz
[i]
[q] During the daytime, when light shines on the [hangman] cells in a leaf, [hangman] pumps are activated. This creates an [hangman] gradient across the membranes of these cells, which pulls in the positive [hangman] ions, and which brings the chloride ions through a [hangman] mechanism.
[c]IGd1YXJk[Qq]
[f]IENvcnJlY3Qh[Qq]
[c]IHByb3Rvbg==[Qq]
[f]IEdvb2Qh[Qq]
[c]IGVsZWN0cm9jaGVtaWNhbA==[Qq]
[f]IEV4Y2VsbGVudCE=[Qq]
[c]IHBvdGFzc2l1bQ==[Qq]
[f]IEdvb2Qh[Qq]
[c]IGNvdHJhbnNwb3J0[Qq]
[f]IEV4Y2VsbGVudCE=[Qq]
[q] The diagram below shows how once water diffuses into the [hangman] of the root (at “i”) it simply follows a path of lower and lower [hangman] potential. Ultimately, water exits through the [hangman] (at “e”) in the lower [hangman](“d”) of the leaf.
[c]IHh5bGVt[Qq]
[f]IENvcnJlY3Qh[Qq]
[c]IHdhdGVy[Qq]
[f]IENvcnJlY3Qh[Qq]
[c]IHN0b21hdGE=[Qq]
[f]IENvcnJlY3Qh[Qq]
[c]IGVwaWRlcm1pcw==[Qq]
[f]IEdvb2Qh[Qq]
[q] The diagram below shows some aspects of water flow in a plant. Where will water proceed after diffusing into the root xylem at “i?”
[textentry single_char=”true”]
[c]IG o=[Qq]
[f]IEV4Y2VsbGVudC4gQWZ0ZXIgdGhlIHJvb3QgeHlsZW0sIHdhdGVyIHdpbGwgcHJvZ3Jlc3MgdG8gdGhlIHh5bGVtIGluIHRoZSBzdGVtLCBhdCAmIzgyMjA7ai4mIzgyMjE7[Qq]
[c]IEVudGVyIHdvcmQ=[Qq]
[f]IE5vLCB0aGF0JiM4MjE3O3Mgbm90IGNvcnJlY3Qu[Qq]
[c]ICo=[Qq]
[f]IE5vLiBBZnRlciB0aGUgcm9vdCB4eWxlbSwgd2F0ZXIgd2lsbCBwcm9ncmVzcyB0byB0aGUgc3RlbSB4eWxlbS4gV2hhdCBsZXR0ZXIgY291bGQgcmVwcmVzZW50IHNvbWV0aGluZyBpbiB0aGUgc3RlbT8=[Qq]
[q] The diagram below shows some aspects of water flow in a plant. After the water is in the stem xylem (at j) you could next expect to find it in
[textentry single_char=”true”]
[c]IG M=[Qq]
[f]IE5pY2UhIEFmdGVyIHRoZSBzdGVtIHh5bGVtLCB3YXRlciB3aWxsIHByb2dyZXNzIHRvIHRoZSB4eWxlbSBpbiB0aGUgbGVhZiwgYXQgJiM4MjIwO2MuJiM4MjIxOw==[Qq]
[c]IEVudGVyIHdvcmQ=[Qq]
[f]IE5vLg==[Qq]
[c]ICo=[Qq]
[f]IE5vLiBBZnRlciB0aGUgc3RlbSB4eWxlbSAoYXQgJiM4MjIwO2omIzgyMjE7KSwgd2F0ZXIgd2lsbCBwcm9ncmVzcyB0byB0aGUgbGVhZiB4eWxlbS4gV2hhdCBsZXR0ZXIgY291bGQgcmVwcmVzZW50IHRoZSB4eWxlbSAoYSB0dWJlKSBpbiB0aGUgbGVhZj8=[Qq]
[q] During the nighttime, the absence of light will cause [hangman] pumps in the [hangman] cells to shut down. As a result, potassium and chloride ions will [hangman] out of these cells. Water will follow by [hangman]. As a result of the loss of water, the [hangman ] will close.
[c]IHByb3Rvbg==[Qq]
[f]IEV4Y2VsbGVudCE=[Qq]
[c]IGd1YXJk[Qq]
[f]IEdyZWF0IQ==[Qq]
[c]IGRpZmZ1c2U=[Qq]
[f]IEdyZWF0IQ==[Qq]
[c]IG9zbW9zaXM=[Qq]
[f]IEdyZWF0IQ==[Qq]
[c]IHN0b21hdGE=[Qq]
[f]IENvcnJlY3Qh[Qq]
[q multiple_choice=”true”] The diagram below represents Strasburger’s experiment on water transport in plants. A severed tree trunk was immersed in a container containing a solution of the plant poison copper sulfate (CuSO4). During the next two weeks, the copper sulfate rose nearly to the top of the plant, killing all the cells it encountered. During the same time period, the volume of the solution in the container decreased by 30 liters. Which of the following is the best explanation of what happened?
[c]IFRoZSBoaWdobHkgbmVnYXRpdmUgd2F0ZXIgcG90ZW50aWFsIGluIHRoZSBDdVNPNA==IHNvbHV0aW9uIHdhcyBhYmxlIHRvIGRyaXZlIHRoZSBzb2x1dGlvbiB1cCBpbnRvIHRoZSBzdGVtIGFuZCBsZWF2ZXMu[Qq]
[f]IE5vLiBXYXRlciBtb3ZlcyBmcm9tIGhpZ2hlciB3YXRlciBwb3RlbnRpYWwgdG8gbG93ZXIgd2F0ZXIgcG90ZW50aWFsLiBJZiB0aGUgd2F0ZXIgcG90ZW50aWFsIGluIHRoZSBzb2x1dGlvbiBpbiB3aGljaCB0aGUgc3RlbSB3YXMgaW1tZXJzZWQgd2FzIGhpZ2hseSBuZWdhdGl2ZSwgdGhlbiB3YXRlciB3b3VsZCBtb3ZlIHRvd2FyZCB0aGUgc29sdXRpb24sIG5vdCBhd2F5IGZyb20gaXQu[Qq]
[c]IA ==TG9zcyBvZiB3YXRlciBmcm9tIHRoZSBsZWF2ZXMgY3JlYXRlZCBhIG5lZ2F0aXZlIHdhdGVyIHBvdGVudGlhbCBpbiB0aGUgbGVhdmVzLCBkcmF3aW5nIHVwIHRoZSBjb3BwZXIgc3VsZmF0ZSBzb2x1dGlvbiB0aHJvdWdoIHRoZSBzdGVtLCBkZWNyZWFzaW5nIHRoZSB2b2x1bWUgb2YgdGhlIGNvcHBlciBzdWxmYXRlIGNvbnRhaW5lci7CoA==[Qq]
[f]IEV4YWN0bHkuIFdoYXQgU3RyYXNidXJnZXIgaGFzIGVzdGFibGlzaGVkIGlzIHRoYXQgd2F0ZXIgaXMgcHVsbGVkIHVwIHRocm91Z2ggYSBwbGFudCBmcm9tIHRoZSBsZWF2ZXMgYWJvdmUgKGFzIG9wcG9zZWQgdG8gYmVpbmcgcHVzaGVkIGZyb20gYmVsb3cpLg==[Qq]
[c]IFRoZSBoeXBvdG9uaWMgc29sdXRpb24gaW4gdGhlIGNvcHBlciBzdWxmYXRlIGNvbnRhaW5lciB3YXMgYWJsZSB0byBtb3ZlIGJ5IG9zbW9zaXMgaW50byB0aGUgcmVzdCBvZiB0aGUgcGxhbnQsIGV2ZW50dWFsbHkgZHJpcHBpbmcgb3V0IHRocm91Z2ggdGhlIGxlYWYgc3RvbWF0YS4=[Qq]
[f]IE5vLiBXaGlsZSB3YXRlciBkb2VzIG1vdmUgZnJvbSBoeXBvdG9uaWMgdG8gaHlwZXJ0b25pYywgdGhlcmUgYXJlIGEgY291cGxlIG9mIHByb2JsZW1zIHdpdGggdGhpcyBjaG9pY2UuIEZpcnN0bHksIHRoZXJlJiM4MjE3O3Mgbm8gZXZpZGVuY2UgdGhhdCB0aGUgY29wcGVyIHN1bGZhdGUgc29sdXRpb24gd2FzIGh5cG90b25pYyB0byB0aGUgZmx1aWRzIGluIHRoZSB4eWxlbS4gU2Vjb25kbHksIG9zbW90aWMgcHJlc3N1cmUgYW5kIGNhcGlsbGFyeSBhY3Rpb24gYXJlIGNhcGFibGUgb2YgbW92aW5nIGEgc29sdXRpb24gb25seSBhIGZldyBjZW50aW1ldGVycyB1cCBhIHN0ZW0u[Qq]
[q] In the diagram below, the leaf xylem is represented by letter
[textentry single_char=”true”]
[c]IG M=[Qq]
[f]IE5pY2Ugam9iLiBUaGUgbGVhZiB4eWxlbSBpcyByZXByZXNlbnRlZCBieSAmIzgyMjA7Yy4mIzgyMjE7[Qq]
[c]IEVudGVyIHdvcmQ=[Qq]
[c]ICo=[Qq]
[f]IE5vLiBIZXJlJiM4MjE3O3MgYSBoaW50LiBUaGUgc3RvbWF0YSBhcmUgb3BlbmluZ3MgaW4gdGhlIGxvd2VyIGVwaWRlcm1pcyBvZiB0aGUgbGVhdmVzLg==[Qq]
[q] In the diagram below, the solute potential is the main force in area number
[textentry single_char=”true”]
[c]ID E=[Qq]
[f]IEdvb2Qgd29yay4gVGhlIG1haW4gY29tcG9uZW50IG9mIHRoZSB3YXRlciBwb3RlbnRpYWwgbW92aW5nIHdhdGVyIGludG8gdGhlIHJvb3QgaXMgc29sdXRlIHBvdGVudGlhbC4gQWZ0ZXIgdGhhdCwgcHJlc3N1cmUgcG90ZW50aWFsIHRha2VzIG92ZXIgYXMgd2F0ZXIgcmlzZXMgZnJvbSB0aGUgcm9vdCB0byB0aGUgc2hvb3Qu[Qq]
[c]IEVudGVyIHdvcmQ=[Qq]
[c]ICo=[Qq]
[f]IE5vLiBIZXJlJiM4MjE3O3MgYSBoaW50LiBMb29rIGZvciBhbiBhcmVhIG9mIHRoZSBwbGFudCB3aGVyZSB3YXRlciBmbG93IHdvdWxkIGJlIGRldGVybWluZWQgYnkgbW92ZW1lbnQgZnJvbSBhIGh5cG90b25pYyBzb2x1dGlvbiB0byBhIGh5cGVydG9uaWMgb25lLg==[Qq]
[q labels = “top”]Connect the values above with the plant regions below.
Region | Ψ (MPa) |
Outside Air | _______ |
Leaf Air Spaces | _______ |
Trunk Xylem | _______ |
Root Xylem | _______ |
Soil Water | _______ |
Values from Principles of Life, p.534 |
[l]-0.3
[fx] No. Please try again.
[f*] Great!
[l]-0.6
[fx] No. Please try again.
[f*] Great!
[l]-1.2
[fx] No. Please try again.
[f*] Correct!
[l]-2.0
[fx] No, that’s not correct. Please try again.
[f*] Great!
[l]-58.5
[fx] No. Please try again.
[f*] Good!
[q]The diagram below shows several possible arrangements of a leaf’s cuticle (its outermost, waxy coating), its mesophyll (the photosynthetically active cells in the middle of a leaf), its stoma (plural of stomata) and air spaces. The arrangement most likely found in a desert plant is
[textentry single_char=”true”]
[c]IE E=[Qq]
[f]IEdvb2Qgd29yay4gJiM4MjIwO0EmIzgyMjE7IHNob3dzIHN0b21hdGEgdGhhdCBhcmUgc3Vua2VuIGludG8gcGl0cywgcmVkdWNpbmcgYWlyZmxvdywgd2hpY2ggd291bGQgcmVkdWNlIHRyYW5zcGlyYXRpb24uIFRoZSB0aGljayBjdXRpY2xlIGlzIGFub3RoZXIgYWRhcHRhdGlvbiBmb3IgcHJldmVudGluZyB3YXRlciBsb3NzLg==[Qq]
[c]IEVudGVyIHdvcmQ=[Qq]
[c]ICo=[Qq]
[f]IE5vLiBIZXJlJiM4MjE3O3MgYSBoaW50LiBUaGluayBhYm91dCB3aGF0IGFkYXB0YXRpb25zIGEgbGVhZiB3b3VsZCBoYXZlIGZvciByZWR1Y2luZyB3YXRlciBsb3NzIGZyb20gdHJhbnNwaXJhdGlvbi4gRm9yIGV4YW1wbGUsIHdvdWxkIHRoZSBjdXRpY2xlIGJlIHRoaW5uZXIgb3IgdGhpY2tlcj8gV291bGQgdGhlIHN0b21hdGEgYmUgbW9yZSBleHBvc2VkIG9yIG1vcmUgcHJvdGVjdGVkIChhbmQgd2hhdCBtaWdodCB0aGF0IGxvb2sgbGlrZSku[Qq]
[q]The diagram below shows several possible arrangements of a leaf’s cuticle (its outermost, waxy coating), its mesophyll (the photosynthetically active cells in the middle of a leaf), its stoma (plural of stomata), and air spaces.
Lily pads are land-living plants that have adapted to living in ponds. Like all terrestrial plants, lily pads need to absorb carbon dioxide from the air.
Which of the leaf arrangements below might be found in a lily pad?
[textentry single_char=”true”]
[c]IG Q=[Qq]
[f]IEF3ZXNvbWUuICYjODIyMDtEJiM4MjIxOyBzaG93cyBzdG9tYXRhIHRoYXQgYXJlIG9uIHRoZSBsZWFmJiM4MjE3O3MgdXBwZXIgc3VyZmFjZS4gVGhpcyBpcyB0aGUgb25seSBzdXJmYWNlIHRoYXQmIzgyMTc7cyBleHBvc2VkIHRvIHRoZSBhaXIsIGFuZCB0aGF0JiM4MjE3O3Mgd2hlcmUgdGhlIHN0b21hdGEgaGF2ZSB0byBiZSBpbiBhIGxpbHkgcGFkIChzaW5jZSB0aGUgbG93ZXIgZXBpZGVybWlzIGlzIGltbWVyc2VkIGluIHdhdGVyKS4=[Qq]
[c]IEVudGVyIHdvcmQ=[Qq]
[c]ICo=[Qq]
[f]IE5vLiBIZXJlJiM4MjE3O3MgYSBoaW50LiBJbiBhIGxpbHkgcGFkLCB0aGUgbGVhdmVzIGFyZSBmbG9hdGluZyBvbiB3YXRlci4gVGhlIGxvd2VyIGVwaWRlcm1pcyBpcyBpbW1lcnNlZCBpbiB3YXRlciwgYW5kIGNhbiYjODIxNzt0IGFic29yYiBjYXJib24gZGlveGlkZS4gV2hlcmUgd291bGQgdGhlIHN0b21hdGEgaGF2ZSB0byBiZT8=[Qq]
[q multiple_choice=”true”]The graph below shows the relationship between transpiration and an unspecified environmental variable. Which one?
[c]dGVtcGVyYXR1cmU=[Qq]
[f]Tm8uIEl0JiM4MjE3O3MgbXVjaCBtb3JlIGxpa2VseSB0aGF0IGluY3JlYXNlZCB0ZW1wZXJhdHVyZSB3b3VsZCBpbmNyZWFzZSByYXRlcyBvZiB0cmFuc3BpcmF0aW9uIChhdCBsZWFzdCBpbml0aWFsbHkpLiBUaGlzIHNob3dzIHRyYW5zcGlyYXRpb24gZmFsbGluZyBhcyB0aGUgdmFyaWFibGUgaW5jcmVhc2VzLiBXaGF0IHZhcmlhYmxlIGNvdWxkIGNhdXNlIHdhdGVyIGxvc3MgdG8gZGVjcmVhc2U/[Qq]
[c]bGlnaHQ=[Qq]
[f]Tm8uIExpZ2h0IGluZHVjZXMgZ3VhcmQgY2VsbHMgdG8gb3BlbiB0aGUgc3RvbWF0YSwgc28geW91JiM4MjE3O2QgZXhwZWN0IGluY3JlYXNlZCBsaWdodCB0byBiZSBhc3NvY2lhdGVkIHdpdGggYW4gaW5jcmVhc2VkIGxldmVsIG9mIHRyYW5zcGlyYXRpb24u[Qq]
[c]aHVtaW RpdHk=[Qq]
[f]RmFidWxvdXMuIEFzIGh1bWlkaXR5IGluY3JlYXNlcywgdGhlIGRpZmZlcmVuY2UgYmV0d2VlbiB0aGUgd2F0ZXIgcG90ZW50aWFsIGluIHRoZSBsZWFmJiM4MjE3O3MgYWlyIHNwYWNlcyBhbmQgdGhlIG91dGVyIGFpciB3aWxsIGRlY3JlYXNlLCB3aGljaCB3aWxsIGRlY3JlYXNlIHRoZSBhbW91bnQgb2YgdHJhbnNwaXJhdGlvbi4=[Qq]
[x][restart]
[/qwiz]
10. A final note about the redwoods
A former student of mine, on a trip to Redwood National Park in northernmost California, learned that the science around redwoods and how they obtain water is changing. Apparently, they’ve evolved mechanisms that enable the leaves in their canopy to directly absorb water from fog. Here’s a link to an explanation in the LA Times. For a peer-reviewed study, click here. I’ll try to better integrate this into a future version of this tutorial.
11. What Now?
Use the menus above to choose another module.