1. Life, Entropy, and Energy Coupling: A review
In Topic 3.4 (ATP and Cell Energy), you learned how the complex order in living things is an inherently unstable condition that can only be maintained by a constant flow of free energy.
If you need to review concepts and terms from that tutorial such as entropy, endergonic, exergonic, free energy, and energy coupling, please follow the link above. Otherwise, take the following quiz.
[qwiz random=”true” qrecord_id=”sciencemusicvideosMeister1961-Energy, Entropy, and Energy Coupling Review (v2.0)”]
[h] Energy, Entropy, and Energy Coupling Review
[i]
[q] The universal tendency of the universe to move from more organized to less organized is called [hangman].
[c]IGVudHJvcHk=[Qq]
[f]IEdvb2Qh[Qq]
[q] [hangman] energy is the type of energy that can be harvested to power work.
[c]IEZyZWU=[Qq]
[f]IEV4Y2VsbGVudCE=[Qq]
[q] In the diagram below, graph 1 shows the course of a(n) [hangman] reaction. This type of reaction [hangman] free energy that can be used to perform [hangman].
[c]IGV4ZXJnb25pYw==[Qq]
[f]IENvcnJlY3Qh[Qq]
[c]IHJlbGVhc2Vz[Qq]
[f]IEV4Y2VsbGVudCE=[Qq]
[c]IHdvcms=[Qq]
[f]IEdvb2Qh[Qq]
[q] In the diagram below, graph 2 shows the course of a(n) [hangman] reaction. In this type of reaction, entropy [hangman], and the products have [hangman] energy than the reactants.
[c]IGVuZGVyZ29uaWM=[Qq]
[f]IENvcnJlY3Qh[Qq]
[c]IGRlY3JlYXNlcw==[Qq]
[f]IEdyZWF0IQ==[Qq]
[c]IG1vcmU=[Qq]
[f]IEV4Y2VsbGVudCE=[Qq]
[q] A reaction like photosynthesis is best represented by which of the graphs below?
[textentry single_char=”true”]
[c]ID I=[Qq]
[f]IFllcy4gUGhvdG9zeW50aGVzaXMgaXMgYW4gZW5kZXJnb25pYyByZWFjdGlvbiBhbmQgaXMgYmVzdCByZXByZXNlbnRlZCBieSBncmFwaCBudW1iZXIgMi4=[Qq]
[c]ICo=[Qq]
[f]IE5vLiBJbiBwaG90b3N5bnRoZXNpcywgdGhlIHByb2R1Y3RzIGhhdmUgbW9yZSBlbmVyZ3kgdGhhbiB0aGUgcmVhY3RhbnRzLiBIb3cgd291bGQgdGhhdCBiZSByZXByZXNlbnRlZD8=[Qq]
[c]IEVudGVyIHRoZSBsZXR0ZXI=[Qq]
[q] Which reaction is not spontaneous and requires an input of energy to run its course (note: ignore activation energy)?
[textentry single_char=”true”]
[c]ID I=[Qq]
[f]IFllcy4gTnVtYmVyIDIgcmVwcmVzZW50cyBhbiBlbmRlcmdvbmljIHJlYWN0aW9uLg==[Qq]
[c]ICo=[Qq]
[f]IE5vLiBIZXJlJiM4MjE3O3MgYSBoaW50IGZvciBuZXh0IHRpbWUuIExvb2sgZm9yIHRoZSByZWFjdGlvbiB3aGVyZSB0aGUgcHJvZHVjdHMgaGF2ZSBtb3JlIGVuZXJneSB0aGFuIHRoZSByZWFjdGFudHMu[Qq]
[c]IEVudGVyIHRoZSBsZXR0ZXI=[Qq]
[q] Cellular respiration is best represented by which of the graphs below?
[textentry single_char=”true”]
[c]ID E=[Qq]
[f]IFllcy4gQ2VsbHVsYXIgcmVzcGlyYXRpb24gaXMgYW4gZXhlcmdvbmljIHJlYWN0aW9uIGFuZCBpcyBiZXN0IHJlcHJlc2VudGVkIGJ5IGdyYXBoIDEu[Qq]
[c]ICo=[Qq]
[f]IE5vLiBIZXJlJiM4MjE3O3MgYSBoaW50IGZvciBuZXh0IHRpbWUuIExvb2sgZm9yIHRoZSByZWFjdGlvbiB3aGVyZSB0aGUgcHJvZHVjdHMgaGF2ZSBsZXNzIGVuZXJneSB0aGFuIHRoZSByZWFjdGFudHMu[Qq]
[c]IEVudGVyIHRoZSBsZXR0ZXI=[Qq]
[q] Which letter represents an endergonic reaction?
[textentry single_char=”true”]
[c]IE I=[Qq]
[f]IFllcy4gJiM4MjIwO0ImIzgyMjE7IHJlcHJlc2VudHMgYW4gZW5kZXJnb25pYyByZWFjdGlvbi4=[Qq]
[c]ICo=[Qq]
[f]IE5vLiBIZXJlJiM4MjE3O3MgYSBoaW50IGZvciBuZXh0IHRpbWUuIExvb2sgZm9yIHRoZSByZWFjdGlvbiB3aGVyZSB0aGUgcHJvZHVjdHMgaGF2ZSBtb3JlIGVuZXJneSB0aGFuIHRoZSByZWFjdGFudHMu[Qq]
[c]IEVudGVyIHRoZSBsZXR0ZXI=[Qq]
[q] Which number represents the source of the energy that makes reaction “B” possible?
[textentry single_char=”true”]
[c]ID E=[Qq]
[f]IFllcy4gJiM4MjIwOzEmIzgyMjE7IHJlcHJlc2VudHMgdGhlIGVuZXJneS1yaWNoIHJlYWN0YW50IHRoYXQmIzgyMTc7cyBtYWtpbmcgcmVhY3Rpb24gJiM4MjIwO0ImIzgyMjE7IHBvc3NpYmxlLg==[Qq]
[c]ICo=[Qq]
[f]IE5vLiBIZXJlJiM4MjE3O3MgYSBoaW50IGZvciBuZXh0IHRpbWUuIFJlYWN0aW9uICYjODIyMDtBJiM4MjIxOyBpcyBjb3VwbGVkIHdpdGggcmVhY3Rpb24gJiM4MjIwO0IuJiM4MjIxOyBXaGF0JiM4MjE3O3MgdGhlIGVuZXJneSBzb3VyY2UgZm9yIHJlYWN0aW9uICYjODIyMDtCLiYjODIyMTs=[Qq]
[c]IEVudGVyIHRoZSBsZXR0ZXI=[Qq]
[q]The diagram below represents the idea of energy [hangman]. Reaction “A” is a(n) [hangman] reaction that’s powering the [hangman] reaction represented by “B.”
[c]Y291cGxpbmc=[Qq]
[c]ZXhlcmdvbmlj[Qq]
[c]ZW5kZXJnb25pYw==[Qq]
[q] In the graphs below, what letter represents activation energy?
[textentry single_char=”true”]
[c]IE Q=[Qq]
[f]IFllcy4gQWN0aXZhdGlvbiBlbmVyZ3kgaXMgcmVwcmVzZW50ZWQgYnkgJiM4MjIwO0QuJiM4MjIxOw==[Qq]
[c]ICo=[Qq]
[f]IE5vLiBIZXJlJiM4MjE3O3MgYSBoaW50IGZvciBuZXh0IHRpbWUuIEZvY3VzIG9uIHRoZSBleGVyZ29uaWMgcmVhY3Rpb24gb24gdGhlIGxlZnQuIElmIHlvdSB3YW50ZWQgdG8gcm9sbCBhIGJhbGwgZG93biBhIGhpbGwsIHlvdSYjODIxNztkIGhhdmUgdG8gZ2l2ZSBpdCBhIGxpdHRsZSBwdXNoIHRvIG92ZXJjb21lIHRoZSBmcmljdGlvbi4gVGhhdCBwdXNoIHJlcXVpcmVzIGEgYml0IG9mIGVuZXJneSwgYmVmb3JlIHRoZSBiYWxsJiM4MjE3O3MgZ3Jhdml0YXRpb25hbCBwb3RlbnRpYWwgZW5lcmd5IGNhbiBiZSBjb252ZXJ0ZWQgdG8ga2luZXRpYyBlbmVyZ3kuIFdoYXQgcGFydCBvZiBncmFwaCAxIGNvdWxkIHJlcHJlc2VudCB0aGF0IHB1c2g/[Qq]
[/qwiz]
2. Autotrophs and heterotrophs
2a. Autotrophs
Autotrophs are organisms that can take low-energy inorganic compounds such as carbon dioxide and water and combine them into high-energy organic compounds such as glucose.
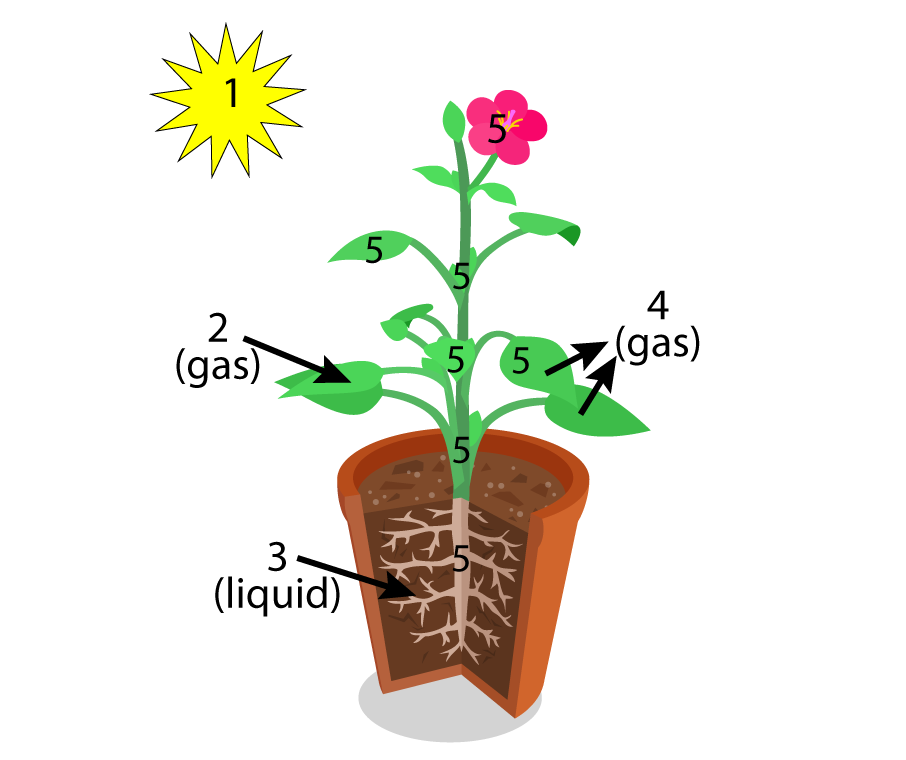
The word “autotroph” means “self-feeder.” The second part of the word — “troph”— comes from the Greek word for nourishment or feeding. The first part —”auto”— means self. An automobile, for example, is a car that drives itself, as opposed to a train car, which has to be pulled by a separate car, the engine.
The autotrophs that we’re most familiar with are plants. Through photosynthesis (an endergonic reaction), plants take in two low-energy compounds — carbon dioxide (2) and water (3). Using the free energy of sunlight (1), plants carry out photosynthesis, creating carbohydrates (5) and releasing oxygen (4).
Because this reaction is powered by light, plants are called photoautotrophs. Other photoautotrophs include algae (such as kelp and other seaweed), a variety of single-celled photosynthetic eukaryotes that are classified as protists, and prokaryotic cyanobacteria.
All of the photoautotrophs mentioned above carry out photosynthesis in a way that produces oxygen:
- CO2 + 2H2O + light energy → [CH2O] + H2O + O2
However, there are some organisms — all bacteria or Archaea — that perform a different form of photosynthesis. Among these organisms, the electron donor is not water, but hydrogen sulfide (H2S):
- CO2 + 2H2S + light energy → [CH2O] + H2O + 2S
Because this type of photosynthesis doesn’t produce oxygen, it’s referred to as anoxygenic photosynthesis.
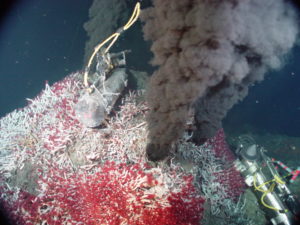
Chemoautotrophs can also synthesize organic compounds from inorganic compounds. However, these organisms don’t use light to boost electrons to a higher energy level so that they can reduce carbon dioxide. Instead, they get their electrons from elemental sulfur, ferrous iron oxide (FeO), hydrogen (H2), or ammonia (NH2).
As we discussed in Topic 7.13, The Origin of Life, Part 4, chemoautotrophic bacteria in Black Smoker vents serve as the basis of a diverse food web. LUCA might have been a bacteria-like chemoautotroph found in an alkaline hydrothermal vent about 3.8 billion years ago.
2b. Heterotrophs
Heterotrophs are organisms that get their energy and matter from other organisms. This can occur through a variety of feeding strategies, such as eating other organisms (like animals), absorbing the dead remains of organisms (like fungi), or parasitizing living organisms (a strategy pursued by animals, fungi, bacteria, and protists). You’re a heterotroph. So are all animals, all fungi, many non-photosynthetic protists, bacteria, archaea, and even a few plants that have evolved into a non-photosynthetic (usually parasitic) niche.
The main metabolic reaction carried out by heterotrophs is cellular respiration:
C6H12O6 + 6O2 → 6CO2 + 6H2O + energy (in the form of ATP)
In addition, a variety of anaerobic fermentations are carried out by heterotrophs.
3. Checking Understanding: Autotrophs and Heterotrophs
[qwiz qrecord_id=”sciencemusicvideosMeister1961-Autotrophs and heterotrophs (v2.0)”]
[h]Autotrophs and heterotrophs
[i]
[q]An organism that can take low-energy inorganic compounds (like [hangman] dioxide and water and combine them into high-energy organic compounds (like glucose) is a(n) [hangman].
[c]Y2FyYm9u[Qq]
[c]YXV0b3Ryb3Bo[Qq]
[q]An organism like a plant that uses light energy to power the creation of high-energy organic compounds is a [hangman].
[c]cGhvdG9hdXRvdHJvcGg=[Qq]
[q]Photoautotrophs create food through [hangman]. In plants, this occurs in organelles called [hangman]. Once the food is created it can be utilized for work through the process of [hangman] [hangman], which occurs mostly in the organelles called [hangman]
[c]cGhvdG9zeW50aGVzaXM=[Qq]
[c]Y2hsb3JvcGxhc3Rz[Qq]
[c]Y2VsbHVsYXI=[Qq]
[c]cmVzcGlyYXRpb24=[Qq]
[c]bWl0b2Nob25kcmlh[Qq]
[q]Organisms that use available chemical energy from sulfur, iron, or other sources to power the creation of high-energy organic compounds are [hangman].
[c]Y2hlbW9hdXRvdHJvcGhz[Qq]
[q]Any organism that gets its energy and matter from other organisms, either by eating or absorption, is a [hangman].
[c]aGV0ZXJvdHJvcGg=[Qq]
[q]Heterotrophs get their energy through cellular [hangman], which occurs primarily in the mitochondria. Heterotrophs can also get energy from organic molecules through an anaerobic process called [hangman], which occurs in the [hangman].
[c]cmVzcGlyYXRpb24=[Qq]
[c]ZmVybWVudGF0aW9u[Qq]
[c]Y3l0b3BsYXNt[Qq]
[x][restart]
[/qwiz]
4. Metabolic rate is a measurement of the flow of energy through an organism
In what follows, we’ll look at how the ongoing flows of energy required to sustain life can be understood quantitatively. To align our discussion with what’s required in an AP Biology course, we’re going to limit our discussion mostly to mammals.
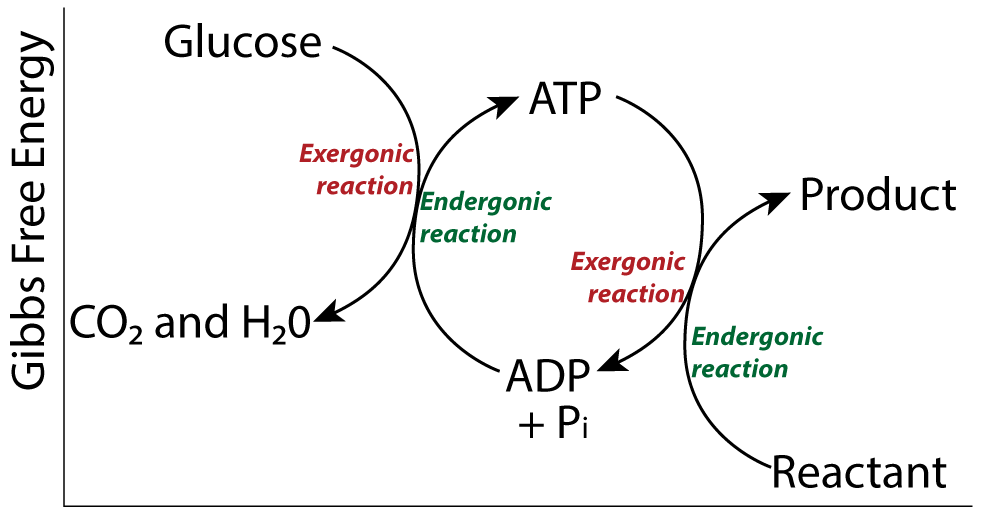
While energy cannot be created or destroyed (the First Law of Thermodynamics) it can change form. In heterotrophs, the chemical potential energy found in food powers cellular respiration, which creates ATP for cellular work. During respiration and during ATP-driven work, chemical energy is transformed into heat energy.
Metabolic rate is the rate at which this transformation — from chemical energy to heat — occurs. But most measurements of metabolic rate measure oxygen consumption. Why? Two reasons. First: cellular respiration involves a direct relationship between oxygen consumption and energy consumption, as shown below.
Food + oxygen → carbon dioxide + water + heat
Second: measuring oxygen consumption is a lot easier than measuring heat production.
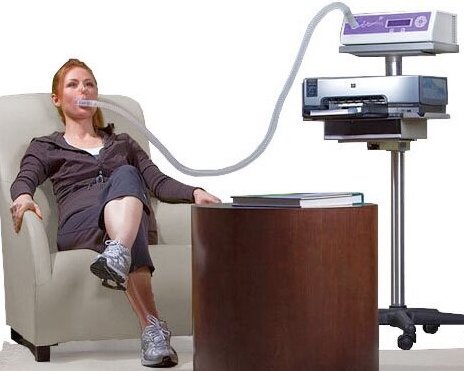
The basal metabolic rate (BMR) is the rate of energy expenditure per unit of time by a mammal resting at a comfortable temperature. Using oxygen as a proxy for energy, BMR is often represented in mL O2/minute. But you’ll see other units as well, such as watts (joules/second), or calories/day (or any other unit of time).
Among mammals, the relationship between metabolic rate and body size remains constant across various shapes, ecological niches, and sizes. Here’s what that looks like when the BMRs of a variety of mammals are plotted on the same graph.
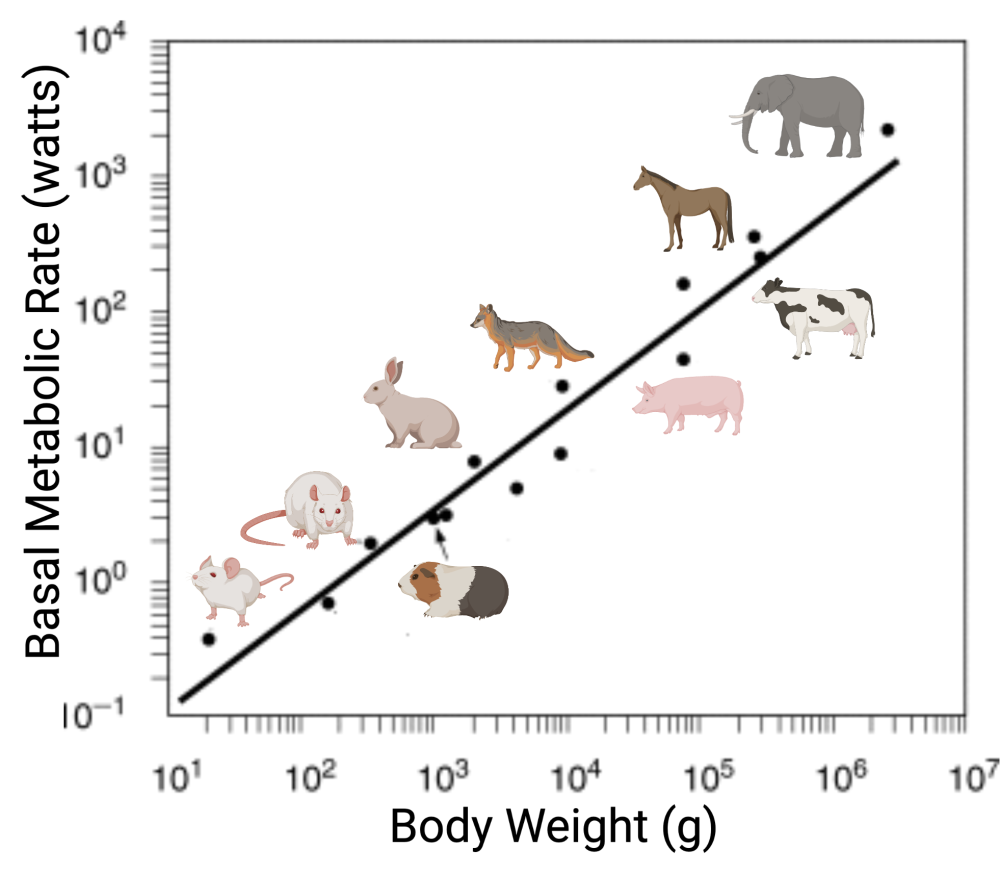
As the body mass of a mammalian species increases, its BMR increases in a proportional way. For example, a mouse, which weighs about 20 grams, consumes about 1/32 the energy of a cat, which weighs 4500 grams.
The proportional increase between body weight and BMR is body mass to the three-quarter power (mass3/4), a relationship known as Kleiber’s law. Though this relation was first formulated in the 1930s, the exact proportion and its underlying causes are still a matter of debate. (Note that Kleiber’s law is beyond the scope of the College Board’s AP Bio course objectives, but if you’re interested, read the Wikipedia article linked above, or this archived article from the New York Times).
The idea that a large mammal is going to consume a lot more oxygen/time than a small mammal seems obvious. But if we take a mammal’s energy requirements/time (its BMR) and divide it by the animal’s mass, we get a very interesting relationship.
Here are a few examples, using daily calorie requirements.
Mammal | Calories/day | Mass (g) | calories/day/gram |
African elephant | 70,000 | 6,000,000 | 0.011 |
Human | 2250 | 62,000 | .036 |
Mouse | 161 | 20 | 8.05 |
Shrew | 144 | 1.8 | 80 |
Shifting from calories/day to the more commonly used measure of metabolic rate — oxygen consumption/time — we get the graph below.
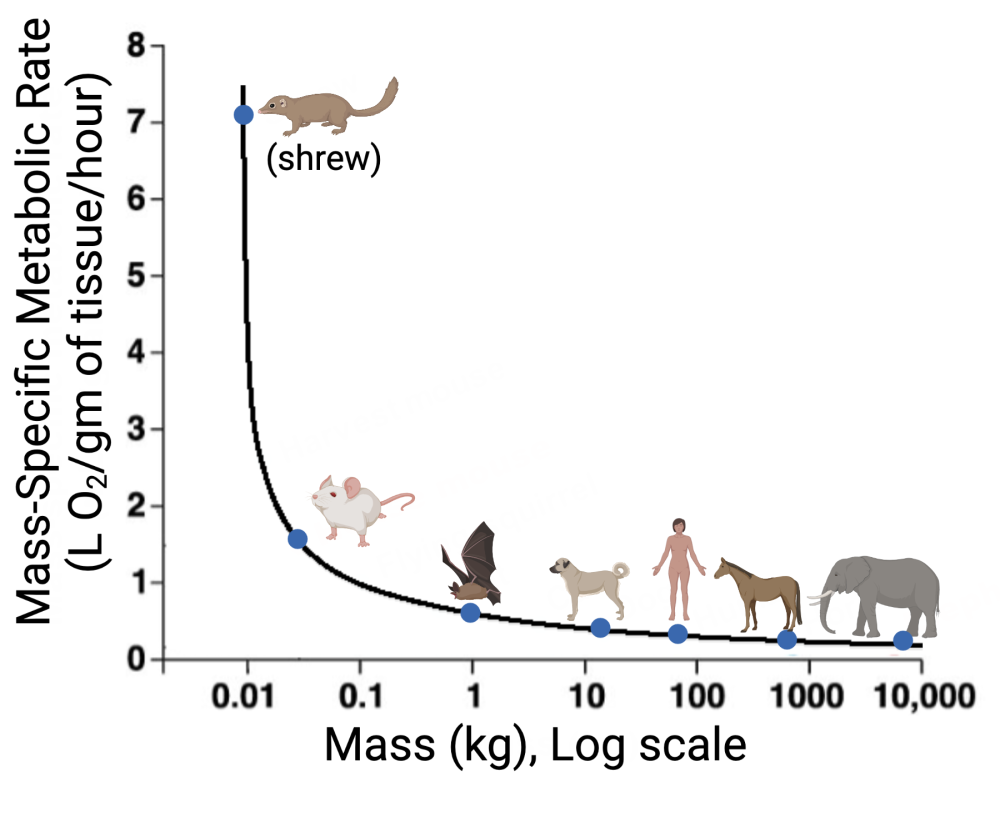
Note the relationship: as mass increases, BMR/gram gets lower and lower. To look at the extremes, note how much less energy a gram of elephant tissue needs than a gram of shrew tissue. Why do elephant cells need so much less energy/time than the cells of smaller mammals? Why does the metabolic rate per gram decrease with size?
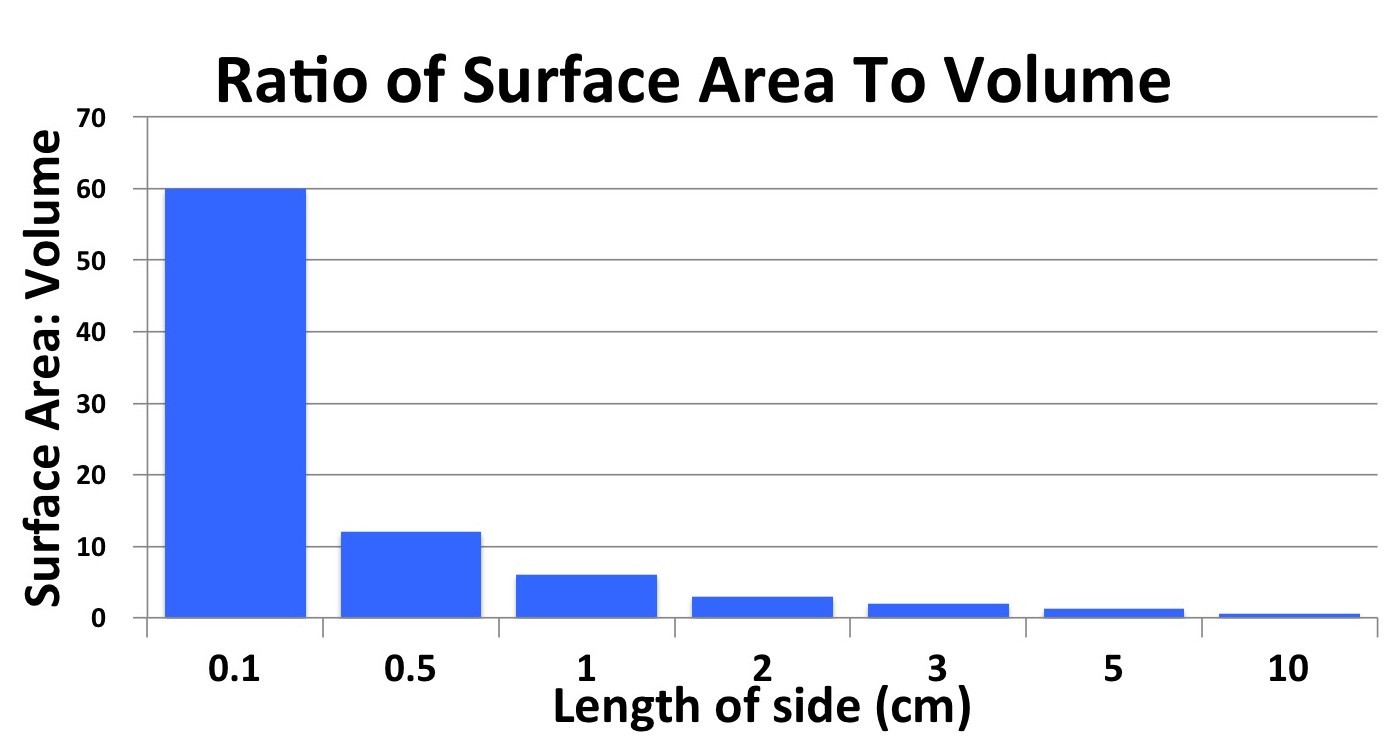
A large part of the answer is surface area to volume ratios. As we learned in AP Bio Topic 2.3, a small object has proportionally much more surface area relative to its volume than a large object (see the diagram on your right).
Because we’re talking about mammals, we can substitute “skin” for surface area. As animals respire, their mitochondria produce a significant amount of heat. That heat can only be removed from the body and dissipated into the external environment through an animal’s skin. Proportionally, an elephant has much less skin/volume than a shrew. So, how does the elephant keep from overheating?
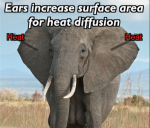
One solution is found in the elephant’s ears, which provide additional surface area for radiating heat into the environment. But that’s not sufficient. The adaptation that large mammals have developed for avoiding overheating is to produce less heat, and they do that by lowering their metabolic rate/gram. If you think of metabolism as the revving of an engine, then imagine that the elephant’s cells are revving much more slowly than the cells of smaller organisms.
Small mammals have the opposite problem. They have a large surface relative to their volume, which means that it’s very easy for them to lose heat. To keep their body temperature at the mammalian homeostatic optimum (about 37°C), they need to increase their metabolic rate so that they produce more heat per gram of tissue. So the corresponding adaptation in small mammals is a much higher metabolic rate. Their cells are revving really fast.
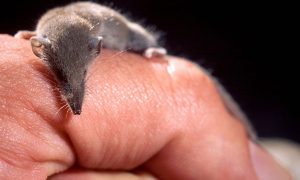
This increase in BMR impacts many processes that occur in the body. The Etruscan shrew, shown to your right perched on someone’s thumb for scale, weighs 1.8 grams (about as much as a large paperclip). Its heart beats 1200 times each minute. It breathes 800 times per minute. If deprived of food, this shrew starves to death in about 4 hours. To keep itself alive it needs to eat 200% of its body weight every day.
The elephant, by contrast, has a heart rate of about 30 beats per minute. It breathes about four or five times per minute. Like other large mammals, it can go for many days without food. It eats about 4% of its body weight every day.
While many physiological processes, such as those listed above, scale by size, many cellular processes don’t. In both elephants and shrews, fundamental processes such as DNA replication, protein synthesis, and diffusion occur at the same rate.
5. Metabolic Rate Flashcards
[qdeck bold_text=”false” qrecord_id=”sciencemusicvideosMeister1961-Metabolic Rate Flashcards (v2.0)”]
[h]Metabolic Rate Flashcards
[i]
[q json=”true” yy=”4″ unit=”8.Ecology” dataset_id=”AP_Bio_Flashcards_2022|12736e209d110″ question_number=”375″ topic=”8.2.Energy_Flow_Through_Ecosystems”] What is metabolic rate? How can it be measured?
[a] An organism’s metabolic rate is the amount of energy that the organism expends during a given amount of time. It is usually measured by rates of oxygen consumption (but can also be measured by rates of carbon dioxide production or heat production).
[q]What’s the basal metabolic rate (BMR)?
[a]The basal metabolic rate (BMR) is the rate of energy expenditure per unit of time by a mammal resting at a comfortable temperature. Using oxygen as a proxy for energy, BMR is often represented in mL O2/minute.
[q json=”true” dataset_id=”AP_Bio_Flashcards_2022|2a44063e5605″ question_number=”376″ unit=”8.Ecology” topic=”8.2.Energy_Flow_Through_Ecosystems”] Among endothermic animals (such as mammals), what’s the relationship between metabolic rate and overall mass?
[a]As body mass increases, the BMR increases proportionally, as shown below.
[q]Among mammals, what’s the relationship between body size and metabolic rate/gram?
[a] Among endothermic animals (for example, mammals) the general rule is that as size increases, relative metabolic rate/gram of tissue decreases. For example, a gram of mouse tissue metabolizes much faster than a gram of elephant tissue. Why? Smaller animals have a larger surface area-to-volume ratio than larger animals. As a result, smaller animals will lose heat more easily than larger animals will. To replace that heat, the smaller animal will need to perform more cellular respiration, increasing its relative metabolic rate.
[q]List some physiological processes that vary with metabolic rate. Using a shrew (the smallest mammal) and an elephant (the largest land mammal), provide some examples.
[a]Processes that vary with metabolic rate include heart rate, breathing rate, and relative food intake. For example, a shrew’s heart beats 1200 times per minute, and it breaths 800 times per minute. An elephant’s heart rate is about 30 beats per minute and it breaths about four or five times per minute.
[q]List some metabolic processes that remain constant, regardless of size.
[a]Some fundamental cellular processes maintain their rate, regardless of the animal’s size. These include DNA replication, protein synthesis, and diffusion.
[x][restart]
[/qdeck]
6. Metabolic Rate Varies Widely Between Endotherms and Ectotherms
The discussion above focused on mammals, which are endotherms. We covered the differences between endotherms and ectotherms in Topic 4.5, Part 1, but here’s a quick review.

Endotherms — a group that’s limited to mammals, birds, and possibly dinosaurs — keep their body temperature constant, regardless of the external temperature. This is shown in line “B” in the graph to your right. The heat that maintains their constant internal temperature is generated from inside their bodies as a by-product of cellular respiration.
In ectotherms, the body temperature conforms to the temperature of the environment (line “A”). Ectotherms include pretty much every living thing that’s not a bird or a mammal. Among the vertebrates, fish, amphibians, and reptiles are ectotherms. All bacteria, archaea, plants, fungi, and invertebrates are also ectotherms.
For the most part, endotherms have much higher metabolic rates than ectotherms. For example, a human being who weighs 81 kilograms (about 180 pounds) requires about 2200 calories of food per day. That’s about 22 slices of bread or an equal number of medium-sized potatoes. An alligator with the same weight would require only about 1/10th of the food energy. In other words, a 180-pound alligator requires the equivalent of two or three slices of bread per day to meet all of its energy needs.
Looking at energy-consumption requirements, being an endotherm seems like a big disadvantage. But the benefit is that a temperature-regulating mammal or bird can stay active at low temperatures. Hence, mammals and birds can survive in cold environments that would be impossible for reptiles, amphibians, and other ectotherms. That’s why Alaska has hundreds of species of endothermic birds and mammals but no ectothermic reptiles. It’s simply too cold for land-living reptiles to survive.
6a. Environmental Challenges and Adaptations in Endotherms
Because endotherms use metabolic energy to keep their body temperatures constant, the relationship between metabolic rate and temperature in endotherms is complex.
At low temperatures, endotherms need to expend energy to raise their body temperature to an optimum level. Shivering, for example, involves muscle contractions that generate heat without generating motion. This high rate of energy expenditure in the cold is shown in the graph on the left as the MMR, which stands for maximum metabolic rate.
As the temperature increases, the amount of energy required to maintain body temperature decreases, until it reaches a TNZ: a thermally neutral zone. That’s the temperature at which minimal energy is required to maintain body temperature, and that is the temperature at which the BMR is measured.
Above that thermally neutral temperature, an endotherm will have to expend energy to cool itself, so the metabolic rate starts to rise.
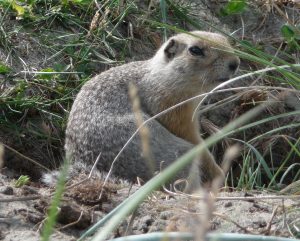
The stress connected with cold temperatures has led to a variety of thermoregulatory adaptations in endotherms. Hibernation is one strategy: by sleeping through the winter, hibernating mammals can avoid the massive energy requirements that they’d experience if they were to remain active. As mammals hibernate, their body temperature can drop considerably. In bears, body temperature can drop from a set point of about 38°C to 31°C. The Arctic ground squirrel hibernates for eight months out of the year, during which time its body temperature drops below freezing (though various adaptations keep these animals from freezing solid, as you can read about here).
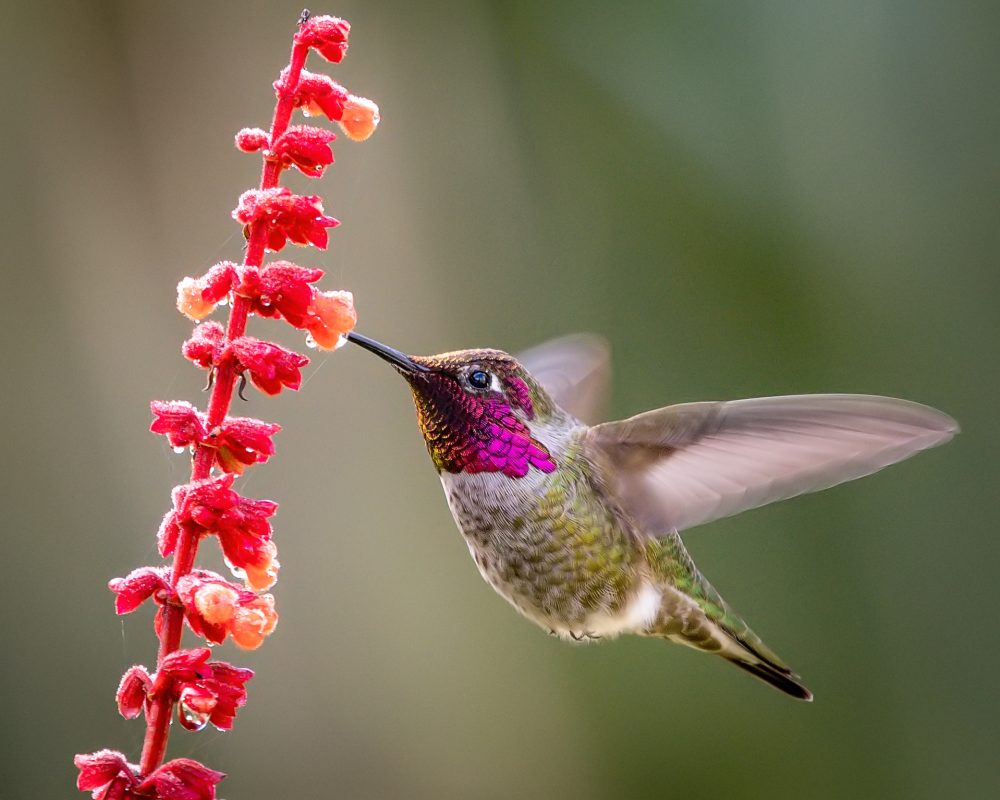
Every night, hummingbirds drop into a state of inactivity called torpor, during which their body temperature can fall by 11°C from their daytime setpoint of 38°C. In a condition called extreme torpor, the body temperature can drop 27 °C.
6b. Adaptations in ectotherms
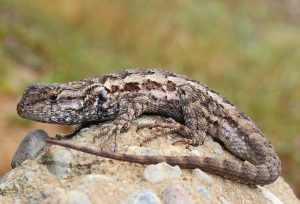
Note that ectotherms aren’t completely at the mercy of the external environment, and have a variety of behavioral strategies to adjust their body temperature to an optimal range. For example, animals such as snakes or lizards will move into the sun to warm up or move into the shade to cool down. When a beehive gets too warm, the bees will spray water over the cells that contain larvae and eggs, and then use their wings to create airflow. The evaporating water cools the hive (in the same way that evaporating sweat cools your body).
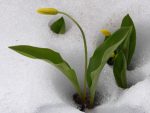
Some plants are thermogenic: they can raise their body temperature above the level of the surrounding air, an adaptation used by some plants to spread their odor and attract pollinators. In other cases, thermogenesis allows plants in cold climates to melt their way through the snow, extending their growing season.
If you haven’t made the connection yet, note that a commonly performed lab from AP Bio Unit 3 was really about ectothermic metabolism. If you need a review, click here. Then come back to finish this section and the material below.

6c. Reproductive Diapause
To end this discussion about metabolic adaptations, let’s look at one shared by both endotherms and ectotherms. Reproductive diapause is a delay in development in response to regular or recurring periods of adverse environmental conditions. For example, in response to drought or temperature extremes, insect larvae might stop developing. This involves storing food molecules and lowering the metabolic rate. When conditions change, indicated by certain stimuli such as temperature change or contact with water, development can resume.
Reproductive diapause occurs throughout the animal kingdom. Some mammal species, after their eggs are fertilized, can delay the implantation of the embryo during adverse conditions. The embryo enters into a dormant state and can be supported with limited energy provided by its mother. When conditions improve, hormonal signals induce implantation and development. Other mammals, including bears, seals, and badgers, have a seasonal diapause. Female bears, for example, breed in late spring/early summer. The embryos remain dormant while the female forages. When her body weight rises to a certain level, hormonal signals induce implantation and hibernation, with the cubs being born in late winter.
7. Flashcards: Endotherms and Ectotherms
[qdeck bold_text=”false” qrecord_id=”sciencemusicvideosMeister1961-Endotherms and Ectotherms Flashcards(v2.0)”]
[h]Endotherms and Ectotherms
[i]
[q] What’s the difference between an ectotherm and an endotherm?
[a] An endotherm is an organism that generates its heat internally through its metabolism. An ectotherm absorbs heat from the environment (for example, from the sun).
[q] Compare and contrast the benefits of being an endotherm or an ectotherm.
[a] Being an endotherm enables an organism to be active regardless of the environmental temperature. That’s why the predominant animals in the Arctic or Antarctic environments are mammals (think of polar bears) and birds (think of penguins). The disadvantage of being an endotherm is that it requires a lot of energy. Ectotherms can survive on about 1/10th of the food energy required by an endotherm of a similar mass.
[q] What are some strategies that ectotherms use to regulate their body temperature?
[a] Ectotherms have a variety of behavioral means of optimizing their body temperature. In larger animals such as lizards and snakes, this includes basking in the sun or resting in the shade. In honeybees, huddling together and moving their wing muscles generates heat that can raise the temperature of the hive in cold weather, or create air currents to cool the hive in warm weather.
[q]What are some strategies that endotherms use to deal with cold stress?
[a]Hibernation is one strategy: by sleeping through the winter, hibernating mammals can avoid the massive energy requirements that they’d experience if they were to remain active and try to forage for food during the lean winter months.
Torpor is a state of inactivity during which body temperature can drop considerably. This is used by birds, bats, and various rodents. Through torpor, all of these endotherms can avoid the energy demands they would experience if they attempted to maintain their body temperature at a constant level.
[q]Explain the relationship between environmental temperature and metabolism in endotherms (like mammals).
[a]
At low temperatures, endotherms need to expend energy to raise their body temperature to an optimum level. As the temperature increases, the amount of energy required to maintain body temperature decreases, until it reaches a thermally neutral zone. That’s the temperature at which minimal energy is required to maintain body temperature. Above the thermally neutral zone, as temperature rises, the metabolic rate rises as the animal has to expend energy to cool itself.
[x]
[q] What is reproductive diapause?
[a] Reproductive diapause is a delay in development in response to regular or recurring periods of adverse environmental conditions.
[restart]
[/qdeck]
8. Checking Understanding: Endotherms and Ectotherms
[qwiz qrecord_id=”sciencemusicvideosMeister1961-Endotherms and Ectotherms Quiz (v2.0)”]
[h]Quiz: Endotherms and Ectotherms
[i]
[q]A(n) [hangman] gets its body heat from the external environment.
[c]ZWN0b3RoZXJt[Qq]
[q]A(n) [hangman] generates its body heat internally, usually as a by product of [hangman] [hangman].
[c]ZW5kb3RoZXJt[Qq]
[c]Y2VsbHVsYXI=[Qq]
[c]cmVzcGlyYXRpb24=[Qq]
[q]Animals that live in cold habitats such as Alaska tend to be [hangman].
[c]ZW5kb3RoZXJtcw==[Qq]
[q]REVIEW: The lentils in the respirometer below are [hangman]. That’s why their [hangman] consumption will increase as the temperature increases.
[c]ZWN0b3RoZXJtcw==[Qq]
[c]b3h5Z2Vu[Qq]
[q]In the graph below, the body temperature of an endotherm is best represented by
[textentry single_char=”true”]
[c]Qg ==
[f]IEV4Y2VsbGVudC4gJiM4MjIwO0ImIzgyMjE7IHNob3dzJiM4MjIxOyBob3cgdGhlIGJvZHkgdGVtcGVyYXR1cmUgb2YgYW4gZW5kb3RoZXJtIHJlbWFpbnMgY29uc3RhbnQsIGRlc3BpdGUgY2hhbmdlcyBpbiB0aGUgdGVtcGVyYXR1cmUgb2YgdGhlIGV4dGVybmFsIGVudmlyb25tZW50Lg==[Qq]
[c]IEVudGVyIHRoZSB3b3Jk[Qq]
[c]ICo=[Qq]
[f]IE5vLiBIZXJlJiM4MjE3O3MgYSBoaW50LiBFbmRvdGhlcm1zIGdlbmVyYXRlIHRoZWlyIGhlYXQgaW50ZXJuYWxseSwgYW5kIHRoZWlyIGJvZHkgdGVtcGVyYXR1cmUgcmVtYWlucyBjb25zdGFudCBkZXNwaXRlIGNoYW5nZXMgaW4gdGhlIGV4dGVybmFsIGVudmlyb25tZW50Lg==[Qq]
[q]Endotherms generally have a much higher [hangman] [hangman] than ectotherms, and correspondingly need to eat much more [hangman] to maintain themselves.
[c]bWV0YWJvbGlj[Qq]
[c]cmF0ZQ==[Qq]
[c]Zm9vZA==[Qq]
[q]Which of the diagrams below shows the relationship between temperature and metabolic rate in an endotherm?
[textentry single_char=”true”]
[c]MQ ==
[f]IEV4Y2VsbGVudC4gJiM4MjIwOzEmIzgyMjE7IHNob3dzJiM4MjIxOyB0aGUgcmVsYXRpb25zaGlwIGJldHdlZW4gdGVtcGVyYXR1cmUgYW5kIG1ldGFib2xpYyByYXRlIGluIGFuIGVuZG90aGVybS4=[Qq]
[c]IEVudGVyIHRoZSB3b3Jk[Qq]
[c]ICo=[Qq]
[f]IE5vLiBIZXJlJiM4MjE3O3MgYSBoaW50LiBFbmRvdGhlcm1zIGhhdmUgdG8gZXhwZW5kIG1vcmUgZW5lcmd5IHRvIG1haW50YWluIHRoZWlyIGJvZHkgdGVtcGVyYXR1cmVzIGF0IGFuIG9wdGltdW0gbGV2ZWwgd2hlbiBpdCYjODIxNztzIGNvbGQu[Qq]
[q]In the diagrams below, what letter shows an endotherm’s basal metabolic rate?
[textentry single_char=”true”]
[c]RA ==
[f]IEV4Y2VsbGVudC4gJiM4MjIwO0QmIzgyMjE7IHNob3dzJiM4MjIxOyBhbiBlbmRvdGhlcm0mIzgyMTc7cyBCTVIu[Qq]
[c]IEVudGVyIHRoZSB3b3Jk[Qq]
[c]ICo=[Qq]
[f]IE5vLiBIZXJlJiM4MjE3O3MgYSBoaW50LiBUaGUgQk1SIGlzIHRoZSBsb3dlc3QgbWV0YWJvbGljIHJhdGUgZm9yIGFuIGVuZG90aGVybS4gRW5kb3RoZXJtcyByZWFjaCB0aGVpciBCTVIgYXQgdGhlIHRoZXJtYWxseSBuZXV0cmFsIHpvbmUu[Qq]
[q]Which of the diagrams below shows the relationship between temperature and metabolic rate in an ectotherm?
[textentry single_char=”true”]
[c]Mg ==
[f]IEV4Y2VsbGVudC4gJiM4MjIwOzImIzgyMjE7IHNob3dzJiM4MjIxOyBhbiBlY3RvdGhlcm0mIzgyMTc7cyBCTVIu[Qq]
[c]IEVudGVyIHRoZSB3b3Jk[Qq]
[c]ICo=[Qq]
[f]IE5vLiBIZXJlJiM4MjE3O3MgYSBoaW50LiBBbiBlY3RvdGhlcm0mIzgyMTc7cyBCTVIgcmlzZXMgYW5kIGZhbGxzIHdpdGggdGhlIHRlbXBlcmF0dXJlIG9mIHRoZSBlbnZpcm9ubWVudC4=[Qq]
[q]Endothermic mammals can reduce their energy requirements during the winter through a prolonged period of sleep known as [hangman], during which their body [hangman] can drop considerably.
[c]aGliZXJuYXRpb24=[Qq]
[c]dGVtcGVyYXR1cmU=[Qq]
[q]A nightly period of inactivity during which body temperature can drop is called [hangman].
[c]dG9ycG9y[Qq]
[q]Ectotherms have a variety of [hangman] adaptations that allow them to modulate their body temperature. For example, to warm themselves, a lizard will bask in the light of the [hangman]. When it’s too warm, a lizard will move into the [hangman].
[c]YmVoYXZpb3JhbA==[Qq]
[c]c3Vu[Qq]
[c]c2hhZGU=[Qq]
[x][restart]
[/qwiz]
9. What’s next?
Proceed to Topic 8.2, Part 2: The Carbon Cycle (the next tutorial in AP Bio Unit 8).