1. Introduction to the Origin of Life
There are only a few science-related questions that are as deep and as meaningful as how did life emerge? The origin of life set the basis for your existence. It began a process that led to us, a self-aware species that can contemplate its own existence, and perhaps, one day, spread awareness to other planets, and even to other stars.
In this tutorial, we’ll look at
- When life arose.
- Some general considerations involved in explaining the origin of life, and
- The key steps involved in life’s origin.
2. When did Life Begin?
Our solar system was formed about 4.6 billion years ago (bya). By about 3.5 bya, life was firmly established on Earth.
How do we know? In Australian sandstone that had been part of a marine shoreline over 3.4 billion years ago, we find microfossils. Unlike the much larger fossils we learned about earlier in this unit, these remains are microscopic, and they can only be seen well with an electron microscope. This sandstone formed as sediments were deposited on the shallow floor of an ancient sea, trapping single-celled, prokaryotic organisms within the sediments.

A team of scientists (Wacey et al.) from Australia and Great Britain collected sandstone from the 3.4 billion-year-old Strelley Pool Formation in Western Australia. Then they cut the sandstone into microscopically thin sections. When viewed under an electron microscope, they saw the cellular structures shown on the left.
When these fossils were chemically analyzed, they revealed two more pieces of evidence for their biological origin. The first is the ratio of carbon-12 to carbon-13. Living things universally prefer to use carbon-12 in their chemical reactions. Wacey et.al found that the samples had 33% to 46% less carbon-13 than would be expected in a sample of non-biological origin, indicating that biological activity had occurred in the creation of these structures. They found a parallel situation in sulfur isotope ratios, leading them to conclude that these cells were sulfur-metabolizing bacteria. (To read the original study, search for “Microfossils of Sulphur-Metabolizing Cells… Wacey” in Google Scholar).

Another piece of evidence for life at 3.4 bya comes from the large structures these early cells created. Some bacteria that live in shallow ocean water create layered, mineralized rock formations called stromatolites. Stromatolites form as photosynthesizing bacteria that live in biofilms on top of rocks in shallow marine waters become covered in sand and other debris. As they reproduce, the new bacteria migrate up to the top of the film, forming a layer of trapped sediment and dead bacterial cells. Year after year, the bacteria keep migrating upward, growing on top of their deceased ancestors. This creates the layered structure shown above and to the right.
If you visit Shark Bay, on the west coast of Australia, you can see living stromatolites (shown below). In terms of evidence for early life, fossilized rock formations with layers thought to be from stromatolites have been found in sedimentary layers that have been dated at 3.4 years old, and, in a disputed finding, even 3.7 billion years old.
Rocks older than about 3.5 bya are generally too deformed by pressure to preserve microfossils. But teams that are studying ratios of carbon 12 to carbon 13 in zircons, graphite-containing crystals, keep pushing the putative date for the origin of life further and further back. Dates for signs of life as old as 4.1 bya have been reported…and disputed. If you’re interested in some of these more tentative findings, you can read this article from Science magazine, or this article in Wikipedia
What’s the takeaway?
- Life emerged about 3.8 billion years ago. With significant fossil evidence from 3.4 bya, it’s clear that life has existed for at least 3.4 billion years and probably for a few hundred million years before that. The scientific consensus – and the date to memorize – is that life emerged about 3.8 billion years ago.
- Life arose about as Early as it could have. The conditions on early Earth were extraordinarily hostile. There were constant asteroid impacts. Volcanic activity was ongoing. But about as soon as Earth was stable enough to allow for a living system to emerge, it emerged. This is a hopeful sign for astrobiologists that are looking for signs of life on other planets. And it raises the question of where on the young Earth life could have arisen — a question we’ll address later in this topic.
3. When Life Began, Checking Understanding
[qwiz random=”true” qrecord_id=”sciencemusicvideosMeister1961-When Life Began (v2.0)”] [h]
When Life Began
[i]
Origin of Life Haiku
A stromatolite
Layers of bacteria
Have turned into stone
[q] The Earth is about [hangman] billion years old.
[c]IDQuNQ==[Qq]
[f]IEdvb2Qh[Qq]
[q] Life first emerged somewhere between 4.1 and 3.5 [hangman] years ago. A consensus view is that life on Earth began at about [hangman] bya.
[c]IGJpbGxpb24=[Qq]
[c]My44[Qq]
[f]IENvcnJlY3Qh[Qq]
[q] Fossilized cells like the ones below are found in remnants of layered bacterial mats called [hangman].
[c]IHN0cm9tYXRvbGl0ZXM=[Qq]
[f]IEdyZWF0IQ==[Qq]
[q] Fossilized cells like the ones below are evident in rocks that are about [hangman] billion years old
[c]IDMuNA==[Qq]
[f]IEdyZWF0IQ==[Qq]
[q]The best description of the microfossils shown below would be
[c]ZXVrYXJ5b3RpYw==[Qq]
[c]cHJva2Fy eW90aWM=[Qq]
[c]bXVsdGljZWxsdWxhcg==[Qq]
[f]Tm8uIFRoZXNlIGZpcnN0IG9yZ2FuaXNtcyB3ZXJlIGFsbW9zdCBjZXJ0YWlubHkgcHJva2FyeW90aWMu[Qq]
[f]RXhjZWxsZW50LiBUaGVzZSBtaWNyb2Zvc3NpbHMgYXJlIHRob3VnaHQgdG8gYmUgc2ltaWxhciB0byBtb2Rlcm4gcGhvdG9zeW50aGV0aWMgYmx1ZS1ncmVlbiBiYWN0ZXJpYSwgYWxzbyBrbm93biBhcyBjeWFub2JhY3RlcmlhLg==[Qq]
[f]Tm8uIFRoaXMgaXMgYSBmaWxhbWVudCBvciBjaGFpbiBvZiBjZWxscywgYnV0IGl0IGRvZXNuJiM4MjE3O3QgcXVhbGlmeSBhcyBhIG11bHRpY2VsbHVsYXIgb3JnYW5pc20u[Qq]
[q]REVIEW: The precise age of ancient igneous (volcanic) rock can be determined through [hangman] dating.
[c]cmFkaW9tZXRyaWM=[Qq]
[q]REVIEW: Radiometric dating is based on the predictable rate of decay of radioactive [hangman].
[c]aXNvdG9wZXM=[Qq]
[q]REVIEW: Isotope M has a half-life of 500 years, during which it decays into isotope N. You’re studying a sample of material that has a relative proportion of 25% M and 75% N. How old is this material?
[c]IDUwMCB5ZWFycyBvbGQu[Qq]
[f]IE5vLiA1MDAgeWVhcnMgaXMgb25lIGhhbGYtbGlmZSwgZHVyaW5nIHdoaWNoIHRpbWUgeW91JiM4MjE3O2QgZXhwZWN0IGhhbGYgb2YgTSB0byBkZWNheSBpbnRvIE4sIGZvciBhIDUwLzUwIHJhdGlvLg==[Qq]
[c]IDc1IHllYXJzLg==[Qq]
[f]IE5vLiBIZXJlJiM4MjE3O3MgaG93IHRvIHRoaW5rIGFib3V0IHRoaXMuIDUwMCB5ZWFycyBpcyBvbmUgaGFsZi1saWZlLCBkdXJpbmcgd2hpY2ggdGltZSB5b3UmIzgyMTc7ZCBleHBlY3QgaGFsZiBvZiBNIHRvIGRlY2F5IGludG8gTiwgZm9yIGEgNTAvNTAgcmF0aW8uIEJhc2VkIG9uIHRoZSBxdWVzdGlvbiwgZmluZCB0aGUgbnVtYmVyIG9mIGhhbGYtbGl2ZXMsIGFuZCBtdWx0aXBseSB0aGF0IGJ5IHRoZSBkdXJhdGlvbiBvZiBhIGhhbGYtbGlmZS4=[Qq]
[c]IDEwMDAgeW VhcnMgb2xk[Qq]
[f]IEV4Y2VsbGVudC4gSWYgb25seSAyNSUgb2YgdGhlIG9yaWdpbmFsIG1hdGVyaWFsIGlzIGxlZnQsIHRoZW4gdHdvIGhhbGYtbGl2ZXMgaGF2ZSBwYXNzZWQsIGZvciBhIHRvdGFsIG9mIDEwMDAgeWVhcnMu[Qq]
[q]In addition to microfossils, evidence for the existence of life about 3.4 billion years ago comes from the [hangman] of isotopes of carbon. The amount of carbon-[hangman] in microfossil samples indicates that the carbon in these fossilized microorganisms is of [hangman] origin.
[c]cmF0aW8=[Qq]
[c]MTI=[Qq]
[c]YmlvbG9naWNhbA==[Qq]
[q]The existence of fossilized bacteria-created rock formations called [hangman] (shown below) from 3.5 billion years ago is additional evidence for the existence of life at that time.
[c]c3Ryb21hdG9saXRlcw==[Qq]
[x][restart]
[/qwiz]
4. Explaining the origin of life: some general considerations
As we saw above, we know that the earliest traces of life in microfossils that date back to 3.5 bya show single-celled organisms with a prokaryotic cell structure. At some point before that, Earth was devoid of life. That leads to two questions:
- How could life have emerged on a non-living planet?
- What were those earliest life forms like?
NASA, which is looking for life on other planets, defines life as “a self-sustaining chemical system capable of Darwinian evolution.”
What would that system be like? At a minimum, it would have to contain the components shown below.
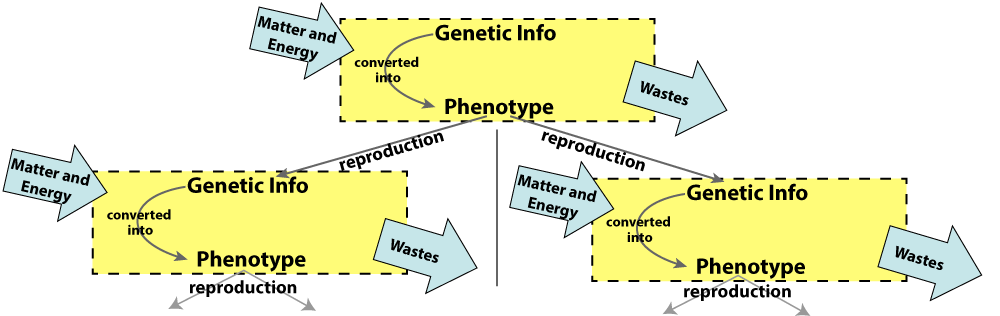
- A chemical means of storing genetic information about itself. In today’s organisms, that’s DNA, but it’s possible to imagine other chemical systems for information storage.
- A way to convert genetic information into a phenotype that can interact with itself and the outside world, so that the system can be reproduced.
- Interacting with itself means replicating its genetic information. Life today uses a team of enzymes for replicating DNA.
- Interacting with the outside world means taking in matter and energy, converting it into the components of the system, and then releasing wastes back into the environment. In organisms today, this is largely done by enzymes which are organized into metabolic pathways.
- Reproduction has to allow for mutation so that these systems can evolve.
The diagram below shows the components of the genotype to phenotype systems that characterize life on Earth.
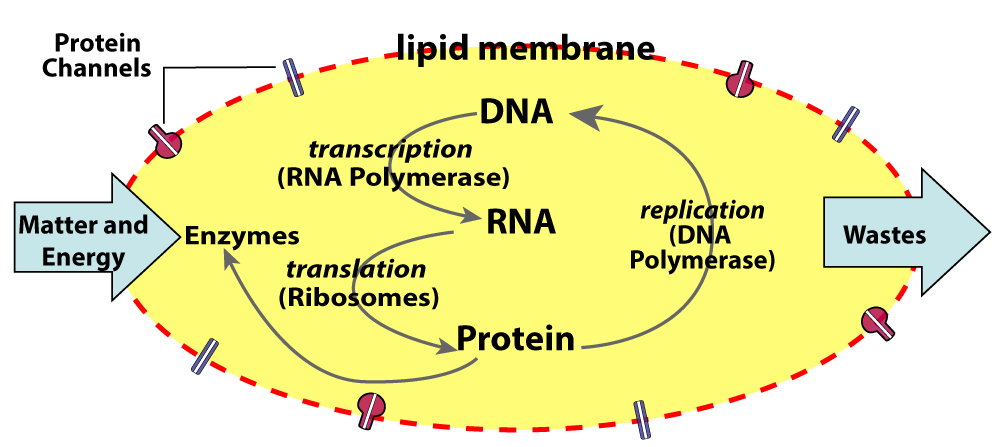
Today, these systems arise as cells reproduce themselves. But at some point, the first self-reproducing genotype-phenotype system had to arise.
It seems unlikely that such a complex system could have arisen at once. What we’re trying to avoid is this:
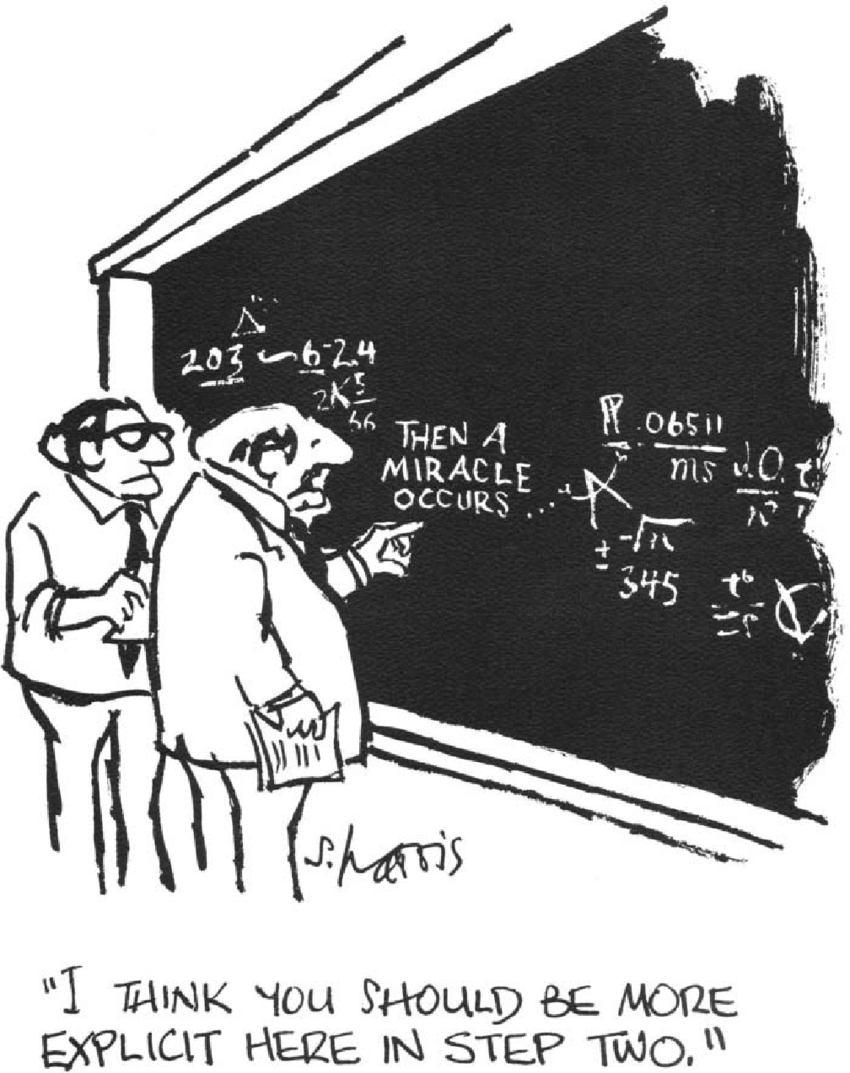
To avoid miracles, origin-of-life researchers have tried to break down the problem of life’s emergence into a series of steps. This series of steps through which non-living matter increases in complexity until a living system emerges is called chemical evolution.
5. Key steps leading to the origin of life
In the diagram below, chemical evolution is shown in steps two and three. The origin of life is at step 4 when the first cells emerge. Steps 5, 6, and 7 involve biological evolution.
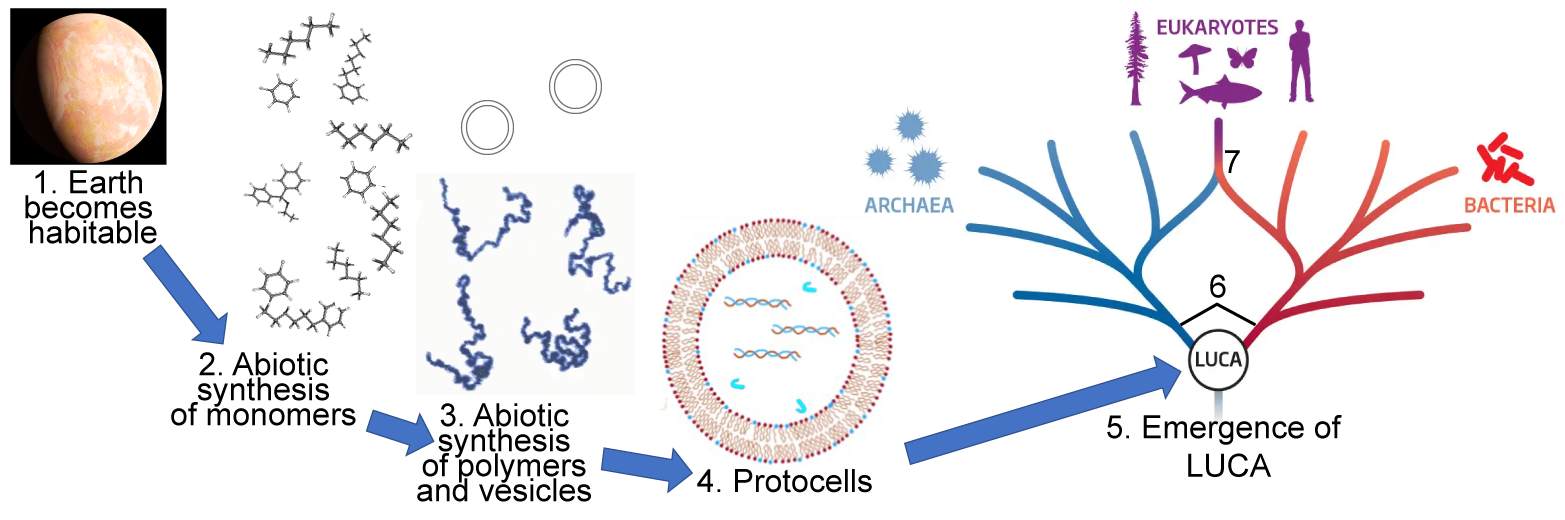
Here are some details about each step.
Step 1: A stable-enough Earth
Earth’s 4.5 billion-year history starts with the Hadean Age. Hades was the Greek underworld, and Hadean Earth was a hellish place:
- It was constantly pummeled by asteroids and comets
- A much closer moon caused massive tides that happened every few hours because the day was much shorter.
As a result of these conditions, the emergence of life has to wait until the planet stabilized. 4.1 billion years ago is thought to be the earliest date when this could have happened.
Step 2: Abiotic formation of monomers
All living things are built from monomers: monosaccharides, amino acids, fatty acids, and nucleotides. Today, all of these monomers are built in plants and other photosynthetic organisms, which create carbohydrates through photosynthesis, and then use these carbohydrates as the starting point for building other monomers. To explain the emergence of life, we need these monomers to arise in the absence of life, or abiotically. In other words, the emergence of monomers (and the polymers that follow) has to happen through chemical evolution.
Step 3: Abiotic synthesis of polymers
All living things today use enzymes (protein polymers) to synthesize polymers from monomers. These polymers are the chemical basis of most of life’s key functions:
- Genetic information storage is based on DNA (a polymer).
- Replication and expression of genetic information are based on DNA polymerase and RNA polymerase, both protein polymers.
- Converting incoming matter and energy into a chemical form that can be used for repair, growth, and reproduction is based on enzymes (protein polymers).
- Controlling what enters and leaves a cell is largely based on membrane channels, which are protein polymers.
For life to emerge, the monomers assembled through chemical evolution need to join together to form polymers. As we’ll see, in any origin of life scenario, this is a difficult step.
Step 4: Formation of protocells
All living things are encapsulated within cells, the outer boundary of which is a lipid membrane. Today, lipids are created by enzymes. So we need an abiotic mechanism for producing lipids, and for bringing these lipids together to form a cell membrane. In addition, we need to explain how genetic material and enzymes can become encapsulated within a lipid membrane.
Once that occurs, we have what’s referred to as a protocell. This protocell might have been quite different from cells today, but once a living, reproducing cell had emerged, biological evolution could take over from chemical evolution.
Step 5: Emergence of LUCA
Further evolution leads to the last universal ancestor of all life, known by the acronym LUCA. LUCA then splits to form the great domains of life.

How do we know what LUCA was like? If we take the shared characteristics of life today — the deep homologies shared by all living things — and simplify them to a bare minimum, we get an organism that has
- A lipid membrane (1 in the diagram to the right) with channels (5) that allow polar and ionic substances to enter and leave the cell.
- A genetic system for survival and reproduction. In today’s organisms, that means
- Genes encoded in DNA (2).
- A genetic code so that these genes can be transcribed into RNA (3).
- Ribosomes that translate RNA into protein (4).
- A system for replicating DNA and passing it on to daughter cells (not shown)
- A system for taking in matter and energy from outside of the cell and using it for repair and reproduction. This is paired with mechanisms for releasing wastes. All organisms use
- protein-based enzymes (6) to convert monomers into polymers and vice versa.
- ATP to perform cellular work. That means there needs to be metabolic machinery such as ATP synthase (7) for creating ATP by chemiosmosis (which is universally used for ATP creation).
6. Quiz: Steps in the Origin of Life; LUCA
[qwiz style=”width: 700px !important; min-height: 450px !important;” qrecord_id=”sciencemusicvideosMeister1961-Steps in the Origin of Life: LUCA (v2.0)”]
[h]Steps in the Origin of Life: LUCA
[i] An origin of life haiku
When life first emerged
Was it heredity first,
Or metabolism?
[q]In the diagram below, which number represents the first polymers?
[textentry single_char=”true”]
[c]ID M=
[f]IEV4Y2VsbGVudC4gTnVtYmVyIDMgcmVwcmVzZW50cyB0aGUgZmlyc3QgcG9seW1lcnMgKGFsb25nIHdpdGggYSBmZXcgdmVzaWNsZXMp[Qq]
[c]ICo=[Qq]
[f]Tm8uIEhlcmUmIzgyMTc7cyBhIGhpbnQuIFBvbHltZXJzIGFyZSBvZnRlbiBsb25nIGNoYWlucyBvZiBtb2xlY3VsZXM=[Qq]
[q]In the diagram below, which number represents a protocell?
[textentry single_char=”true”]
[c]ID Q=
[f]IE5pY2UuIE51bWJlciA0IHJlcHJlc2VudHMgYSBwcm90b2NlbGw6IGVzc2VudGlhbGx5IHRoZSBmaXJzdCBsaXZpbmcgb3JnYW5pc20u[Qq]
[c]ICo=[Qq]
[f]Tm8uIEhlcmUmIzgyMTc7cyBhIGhpbnQuIEZpbmQgYSBsaXBpZCBiaWxheWVyIHdpdGggc29tZSBnZW5ldGljIG1hdGVyaWFsIGluc2lkZSBvZiBpdC4=[Qq]
[q]In the diagram below, which number represents the first monomers?
[textentry single_char=”true”]
[c]ID I=
[f]IEF3ZXNvbWUuIE51bWJlciAyIHJlcHJlc2VudHMgdGhlIGZpcnN0IG1vbm9tZXJzLg==[Qq]
[c]ICo=[Qq]
[f]Tm8uIEhlcmUmIzgyMTc7cyBhIGhpbnQuIE1vbm9tZXJzIGFyZSByZWxhdGl2ZWx5IHNtYWxsIG1vbGVjdWxlcyBjb21wb3NlZCBvZiBhIGRvemVuIG9yIGEgZmV3IGRvemVuIGF0b21zLg==[Qq]
[q]In the diagram below, the split that led to Archaea and Bacteria is represented by…
[textentry single_char=”true”]
[c]ID Y=
[f]IEdvb2Qgd29yay4gTnVtYmVyIDYgcmVwcmVzZW50cyB0aGUgc3BsaXQgYmV0d2VlbiBiYWN0ZXJpYSBhbmQgYXJjaGFlYS4=[Qq]
[c]ICo=[Qq]
[f]Tm8uIEhlcmUmIzgyMTc7cyBhIGhpbnQuIEZpbmQgTFVDQSwgYW5kIHRoZW4gZmluZCB3aGVyZSBpdCBzcGxpdHMgaW50byB0d28gYnJhbmNoZXMu[Qq]
[q]In the diagram below, which number represents the uptake of what would become the ancestor of mitochondria by an Archaeal cell?
[textentry single_char=”true”]
[c]ID c=
[f]IEF3ZXNvbWUuIE51bWJlciA3IHJlcHJlc2VudHMgdGhlIG9yaWdpbiBvZiB0aGUgZXVrYXJ5b3Rlcywgd2hpY2ggaGFwcGVuZWQgYnkgYW4gZW5kb3N5bWJpb3RpYyBtZXJnZXIgb2YgYW4gQXJjaGFlYWwgY2VsbCB0aGF0IHRvb2sgdXAgYSBiYWN0ZXJpYWwgY2VsbC4gVGhlIGxhdHRlciBldm9sdmVkIGludG8gYSBtaXRvY2hvbmRyaW9uLg==[Qq]
[c]ICo=[Qq]
[f]Tm8uIEhlcmUmIzgyMTc7cyBhIGhpbnQuIFRoZSBFdWthcnlvdGVzIGFyb3NlIHRocm91Z2jCoA==YW4gZW5kb3N5bWJpb3RpYyBtZXJnZXIgb2YgYW4gQXJjaGFlYWwgY2VsbCB0aGF0IHRvb2sgdXAgYSBiYWN0ZXJpYWwgY2VsbC4gVGhlIGxhdHRlciBldm9sdmVkIGludG8gYSBtaXRvY2hvbmRyaW9uLsKg[Qq]
[q labels= “top”]The table below shows some key steps involved in the origin of life. Use what you learned above (and trial and error) to figure it out.
FIRST | Abiotic creation of _____________ (the molecular building blocks of life) | |||
NEXT | Abiotically link monomers to form ___________ | Origin of ______________. Create a self-perpetuating system for processing matter and _________ and for removing wastes. | Origin of _____________. Provide the system with a way to pass on ______________ for maintenance, growth, and reproduction | Encapsulate the system with a _____________ to keep it from dissolving away, creating the first primitive _______. |
[l]cells
[f*] Correct!
[fx] No, that’s not correct. Please try again.
[l]energy
[f*] Correct!
[fx] No, that’s not correct. Please try again.
[l]heredity
[f*] Excellent!
[fx] No. Please try again.
[l]instructions
[f*] Great!
[fx] No. Please try again.
[l]metabolism
[f*] Excellent!
[fx] No. Please try again.
[l]membrane
[f*] Great!
[fx] No. Please try again.
[l]monomers
[f*] Great!
[fx] No, that’s not correct. Please try again.
[l]polymers
[f*] Good!
[fx] No, that’s not correct. Please try again.
[q labels= “top”]Here’s the same table, but with different blanks to fill in.
FIRST | __________ creation of monomers (the molecular__________ blocks of life. | |||
NEXT | Abiotically link _____________ to form polymers | Origin of metabolism. Create a self-_______________ system for processing ________ and energy and for removing ________ | Origin of heredity. Provide the system with a way to ___________ instructions about maintenance, growth, and _______________. | ___________ the system with a membrane to keep it from dissolving away, creating the first primitive cells. |
[l]Abiotic
[f*] Good!
[fx] No, that’s not correct. Please try again.
[l]building
[f*] Correct!
[fx] No, that’s not correct. Please try again.
[l]Encapsulate
[f*] Correct!
[fx] No, that’s not correct. Please try again.
[l]matter
[f*] Correct!
[fx] No, that’s not correct. Please try again.
[l]monomers
[f*] Great!
[fx] No. Please try again.
[l]pass on
[f*] Great!
[fx] No, that’s not correct. Please try again.
[l]perpetuating
[f*] Great!
[fx] No. Please try again.
[l]reproduction
[f*] Great!
[fx] No. Please try again.
[l]wastes
[f*] Correct!
[fx] No. Please try again.
[q] And here’s that table once more in a fill-in-the-blanks format.
FIRST | [hangman] creation of monomers (the molecular building blocks of life) | |||
NEXT | Abiotically link monomers to form [hangman] | Origin of [hangman]. Create a system for processing matter and energy and for removing wastes. | Origin of [hangman]. Provide the system with a way to pass on instructions for maintenance, growth, and reproduction | Encapsulate the system with a [hangman] to keep it from dissolving away, creating the first primitive [hangman]. |
[c]IGFiaW90aWM=[Qq]
[f]IEV4Y2VsbGVudCE=[Qq]
[c]IHBvbHltZXJz[Qq]
[f]IEV4Y2VsbGVudCE=[Qq]
[c]IG1ldGFib2xpc20=[Qq]
[f]IEV4Y2VsbGVudCE=[Qq]
[c]IGhlcmVkaXR5[Qq]
[f]IENvcnJlY3Qh[Qq]
[c]IG1lbWJyYW5l[Qq]
[f]IEV4Y2VsbGVudCE=[Qq]
[c]IGNlbGxz[Qq]
[f]IEV4Y2VsbGVudCE=[Qq]
[q multiple_choice=”true”] Based on what you know about biology, the first self-replicating informational molecule would probably be a(n) __________ acid.
[c]IGFtaW5v[Qq]
[f]IE5vLiBBbWlubyBhY2lkcyBhcmUgdGhlIG1vbm9tZXJzIG9mIHByb3RlaW5zLiBUaGV5JiM4MjE3O3JlIG5vdCBpbmZvcm1hdGlvbmFsLg==[Qq]
[c]IG51Y2 xlaWM=[Qq]
[f]IEV4Y2VsbGVudC4gSW4gbGlmZSB0b2RheSwgaW5mb3JtYXRpb25hbCBtb2xlY3VsZXMgYXJlIG51Y2xlaWMgYWNpZHM6IGVpdGhlciBETkEgb3IgUk5BLg==[Qq]
[c]IGZhdHR5[Qq]
[f]IE5vLiBGYXR0eSBhY2lkcyBhcmUgY29tcG9uZW50cyBvZiBsaXBpZHMuIFRoZXkmIzgyMTc7cmUgbm90IHVzZWQgZm9yIGluZm9ybWF0aW9uLCBhbmQgaXQmIzgyMTc7cyBoYXJkIHRvIHNlZSBob3cgdGhleSBjb3VsZCBiZS4=[Qq]
[q multiple_choice=”true”] From the choices below, the type of molecule that would make up an encapsulating membrane would probably be primarily composed of _________ acids.
[c]IGFtaW5v[Qq]
[f]IE5vLiBBbWlubyBhY2lkcyBhcmUgdGhlIG1vbm9tZXJzIG9mIHByb3RlaW5zLiBUaGV5IGFyZSBjcnVjaWFsbHkgaW1wb3J0YW50IHBhcnRzIG9mIG1lbWJyYW5lcywgYnV0IHRoZXkmIzgyMTc7cmUgbm90IHRoZSBtYWluIHN0cnVjdHVyYWwgY29tcG9uZW50IG9mIG1lbWJyYW5lcy4=[Qq]
[c]IG51Y2xlaWM=[Qq]
[f]IE5vLiBJbiBsaWZlIHRvZGF5LCBpbmZvcm1hdGlvbmFsIG1vbGVjdWxlcyBhcmUgbnVjbGVpYyBhY2lkcy4gWW91JiM4MjE3O3JlIGxvb2tpbmcgZm9yIGEgbW9sZWN1bGUgdGhhdCB3b3VsZCBtYWtlIHVwIG9uZSBvZiB0aGUgY29tcG9uZW50cyBvZiBtZW1icmFuZXMu[Qq]
[c]IGZh dHR5[Qq]
[f]IEV4Y2VsbGVudC4gRmF0dHkgYWNpZHMgYXJlIGEga2V5IGNvbXBvbmVudCBvZiB0aGUgbW9sZWN1bGVzIHRoYXQgbWFrZSB1cCBtZW1icmFuZXMgKHBob3NwaG9saXBpZHMgaW4gYmFjdGVyaWEgYW5kIGV1a2FyeWEsIGEgcmVsYXRlZCBsaXBpZCBtb2xlY3VsZSBpbiBhcmNoYWVhKS4=[Qq]
[q]In the diagram below, which number represents chemiosmosis
[textentry single_char=”true”]
[c]ID c=
[f]IEV4Y2VsbGVudC4gTnVtYmVyIDcgcmVwcmVzZW50cyB0aGUgY2hlbWlvc21vdGljIHByb2R1Y3Rpb24gb2YgQVRQIHZpYSB0aGUgQVRQIHN5bnRoYXNlIGNoYW5uZWwu[Qq]
[c]ICo=[Qq]
[f]Tm8uIEhlcmUmIzgyMTc7cyBhIGhpbnQuIENoZW1pb3Ntb3NpcyBpbnZvbHZlcyB0aGUgZmFjaWxpdGF0ZWQgZGlmZnVzaW9uIG9mIGh5ZHJvZ2VuIGlvbnMgdG8gcG93ZXIgQVRQIHN5bnRoZXNpcy4=[Qq]
[q]In the diagram below, which number represents translation?
[textentry single_char=”true”]
[c]ID Q=
[f]IEV4Y2VsbGVudC4gTnVtYmVyIDQgc2hvd3MgYSByaWJvc29tZSB0cmFuc2xhdGluZyBtUk5BIGludG8gcHJvdGVpbi4=[Qq]
[c]ICo=[Qq]
[f]Tm8uIEhlcmUmIzgyMTc7cyBhIGhpbnQuIFRyYW5zbGF0aW9uIGlzIHByb3RlaW4gc3ludGhlc2lzLCBhbmQgaXQmIzgyMTc7cyBjYXJyaWVkIG91dCBieSByaWJvc29tZXMu[Qq]
[q]The diagram below shows the array of features that are thought to have been present in [hangman].
[c]TFVDQQ==[Qq]
[x]
[restart]
[/qwiz]
7. Time makes improbable events possible
One idea to hold in mind as you think about the probability of chemical evolution leading up to the emergence of the first cells is the vast amount of time during which this process occurred.
Let’s say that the Earth became stable enough for life 4.1 billion years ago, and that life emerged 3.8 billion years ago. In between is a timespan of 300 million years. Even if the sequence of events leading to a “self-sustaining chemical system capable of Darwinian evolution” (NASA’s definition of life) was extraordinarily unlikely, there was an enormous amount of time for this sequence to occur. An unlikely event with a 1-in-a-million probability played out over a million years becomes a likely event.
In addition, because of the conditions on early Earth, chemical evolution was probably occurring in multiple spots around the planet, increasing the probability that somewhere on Earth, the right pieces would assemble to produce the genotype-phenotype systems we described above.
8. What’s Next?
Proceed to Topic 7.13, Part 2: Abiotic Formation of Monomers and the Miller-Urey Experiment, the next tutorial in Topic 7.13, The Origin of Life.