1. Introduction: What is Homeostasis?
Imagine, as in the image shown on the left, that you were trying to balance yourself on a board. You’d be adjusting your weight to the left and to the right. Each moment, your legs and arms would shift position. If you were outdoors, and the wind blew from your left, you might compensate by leaning to the right.
That process of trying to maintain your balance is the essence of homeostasis: the tendency of a living system to maintain its internal conditions at a relatively constant, optimal level. (Optimal means “best or most favorable”).
Homeostasis, like balancing on a board, involves work. When a change in an organism’s internal or external environment moves the system away from an optimal level, the organism needs to adjust conditions so that they return to the optimal level. These optimal levels are called set points. Here are a few examples.
- For mammals like us, the body temperature set point fluctuates around 37°C.
- Our blood’s pH is slightly alkaline, with a set point between pH 7.35 and pH 7.45.
- Our blood glucose level fluctuates. It rises immediately after eating, and then a variety of homeostatic mechanisms lower it to what’s called the “fasting level.” At that point (several hours after eating) the set point is between 70 and 100 mg/100 mL. If your fasting blood glucose level is about 100 mg/100 mL you might be at risk for diabetes.
Keeping these conditions (and many others) within a healthy, life-sustaining range is what homeostasis is all about. Let’s see how it works.
2. Body Temperature Regulation: Regulators and Conformers, Ectotherms and Endotherms
2a. Regulating and Conforming
The first thing to note is that not all organisms regulate all internal conditions to the same degree. Look at this graph showing the body temperature of a snake (A) and a coyote (B). As the external temperature rises and falls, so does the snake’s internal temperature. That makes the snake, in terms of body temperature, a conformer. Its body temperature conforms to the temperature of the external environment.
By contrast, the coyote, in terms of body temperature, is a regulator. Whether the external temperature is high or low, the coyote (like all mammals) works to keep its body temperature at a constant 37°C.
Note that both strategies have benefits and drawbacks. It takes work to regulate. In the case of body temperature regulation, that work has to be fueled by food energy. As a result, for each kilogram of body weight, the daily energy requirements of a mammal are much higher than the daily energy requirements of a reptile. For example, a human being who weighs 81 kilograms (about 180 lbs) requires about 2700 calories of food per day. That’s about 27 slices of bread or an equal number of medium-sized potatoes. An alligator with the same weight would require only about 1/10th of the food energy. In other words, a 180 lb alligator requires the equivalent of two or three slices of bread to meet all of its energy needs.
Looking at energy-consumption requirements, being a temperature regulator seems like a big disadvantage. But the benefit is that a temperature-regulating mammal can stay active at low temperatures. Hence, mammals can survive in cold environments that would be impossible for reptiles. That’s why Alaska has hundreds of species of birds and mammals (both body-temperature regulators) but no land-living reptiles (such as snakes or lizards). It’s simply too cold for land-living reptiles to survive.
In terms of body temperature regulation, there are two strategies to know about.
2b. Ectotherms
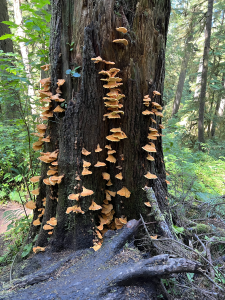
Ectotherms are organisms that get heat from the external environment. Most ectotherms are conformers, and their body temperature fluctuates with the temperature of the environment.
Plants, fungi, and bacteria are ectothermic.
Possibly, you might have observed this yourself in your AP Bio laboratory. The device below is a respirometer, and it measures the oxygen consumption rate of whatever organism you place inside of it. The respirometer below contains geminating lentils. Imagine an experiment in which one group of lentils is in a cold environment, and a second group is in a warm environment. The lentils’ internal temperature would come to match the temperature of their environment. As a result, the metabolic rate of the cold lentils would be much lower than that of the warm lentils, which you could observe by comparing the oxygen consumption rate in the two groups. To watch a video about how respirometers work, click here.
Most animals are ectothermic, too. All of the invertebrates (animals without backbones) are ectotherms, including arthropods (such as insects or crabs), mollusks (clams, snails, octopi, and squids), and echinoderms (seastars, sea urchins, and sand dollars). Among the vertebrates (animals with backbones) fish, amphibians, and most reptiles are ectotherms.
Note that ectotherms aren’t completely at the mercy of the external environment, and have a variety of strategies to adjust their body temperature to an optimal range. For example, animals such as snakes or lizards will move into the sun to warm up or move into the shade to cool down. When a beehive gets too warm, the bees will spray water over the cells that contain larvae and eggs, and then use their wings to create airflow. The evaporating water cools the hive (in the same way that evaporating sweat does, as we’ll see below). Thermogenic plants can raise their body temperature above the level of the surrounding air, an adaptation used by some plants to spread their odor and attract pollinators.
2c. Endotherms
Endotherms generate their heat internally, mostly as a result of heat generated during cellular respiration. Endothermy (being an endotherm) is mostly limited to two existing groups of animals: mammals and birds. It’s also widely thought that dinosaurs were endothermic (but controversy remains, as you can read here).
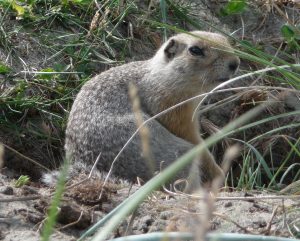
Note that while mammals and birds are both endothermic conformers, there are many energy-saving adaptations found among members of both groups. Hibernation is one strategy: by sleeping through the winter, hibernating mammals can avoid the massive energy requirements that they’d experience if they were to remain active. As mammals hibernate, their body temperature can drop considerably. In bears, body temperature can drop from a set point of about 38°C to 31°C. The Arctic ground squirrel hibernates for eight months out of the year, during which time its body temperature drops below freezing (though various adaptations keep these animals from freezing solid, as you can read about here).
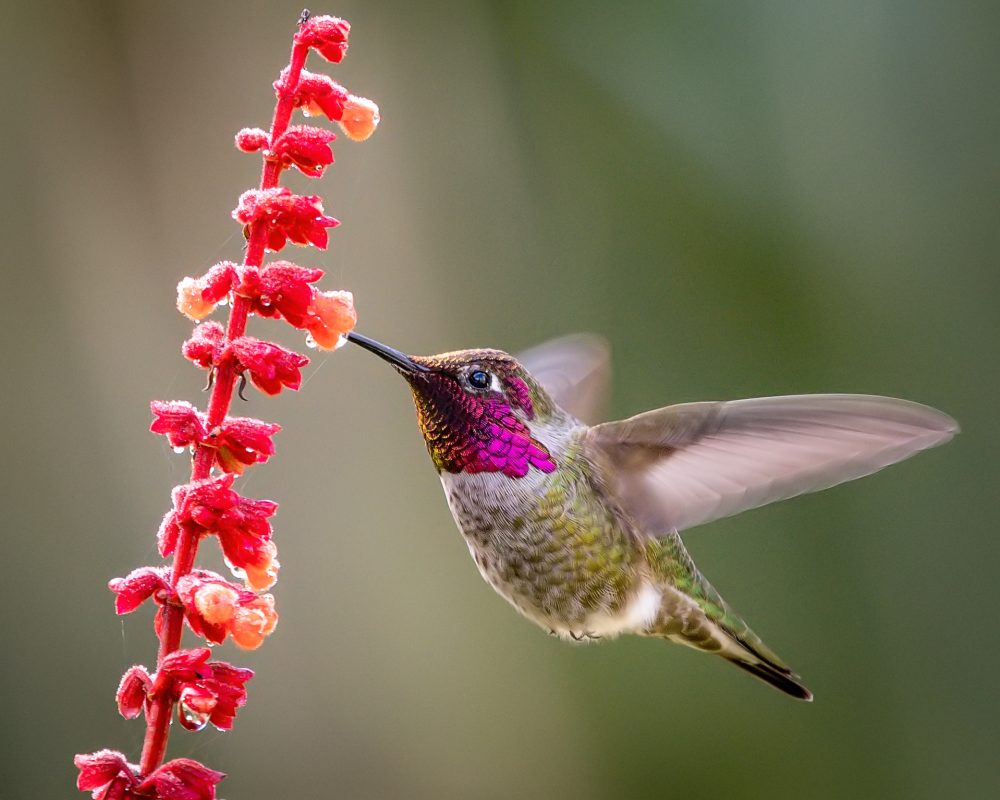
Every night, hummingbirds drop into a state of inactivity called torpor, during which their body temperature can fall by 11°C from their daytime setpoint of 38°C. In a condition called extreme torpor, the body temperature can drop 27 °C.
2d. What about Cold Blooded and Warm-Blooded?
Ectotherms, particularly ectothermic animals like reptiles and amphibians are commonly referred to as being cold-blooded. That’s because a salamander or snake that you’d pick up off of the forest floor will feel cold: it’ll be the same temperature as the cool forest floor. However, these animals aren’t always cold. The body temperature of a lizard basking in the sun or a rattlesnake in the desert during the daytime can approach or even exceed the body temperature of an endothermic mammal.
As we’ve seen, endotherms like birds and mammals aren’t always warm-blooded (a term frequently used to describe these animals). For energy conservation, many birds and mammals allow their body temperature to significantly drop during nighttime torpor or winter hibernation.
3. Checking Understanding: Homeostasis, Regulators, Conformers, Endotherms, Ectotherms
[qwiz qrecord_id=”sciencemusicvideosMeister1961-Homeostasis, Endotherms, Ectotherms (v2.0)”]
[h]Homeostasis, Regulators, Conformers, Endotherms, Ectotherms.
[i]
[q] The tendency of a living system to maintain internal stability, even in the face of external change, is called [hangman].
[c]IGhvbWVvc3Rhc2lz[Qq]
[q]A [hangman] allows an internal variable to rise and fall as the outside environment changes.
[c]Y29uZm9ybWVy[Qq]
[q]A [hangman] works to maintain an internal condition in a constant range, despite environmental changes.
[c]cmVndWxhdG9y[Qq]
[q]A(n) [hangman] gets its body heat from the external environment.
[c]ZWN0b3RoZXJt[Qq]
[q]A(n) [hangman] generates its body heat internally, usually as a byproduct of [hangman] [hangman].
[c]ZW5kb3RoZXJt[Qq]
[c]Y2VsbHVsYXI=[Qq]
[c]cmVzcGlyYXRpb24=[Qq]
[q]Animals that live in cold habitats such as Alaska tend to be [hangman].
[c]ZW5kb3RoZXJtcw==[Qq]
[q]The lentils in the respirometer below are [hangman]. That’s why their [hangman] consumption will increase as the temperature increases.
[c]ZWN0b3RoZXJtcw==[Qq]
[c]b3h5Z2Vu[Qq]
[q]In the graph below, the body temperature of an endotherm is best represented by
[textentry single_char=”true”]
[c]Qg ==
[f]IEV4Y2VsbGVudC4gJiM4MjIwO0ImIzgyMjE7IHNob3dzJiM4MjIxOyBob3cgdGhlIGJvZHkgdGVtcGVyYXR1cmUgb2YgYW4gZW5kb3RoZXJtIHJlbWFpbnMgY29uc3RhbnQsIGRlc3BpdGUgY2hhbmdlcyBpbiB0aGUgdGVtcGVyYXR1cmUgb2YgdGhlIGV4dGVybmFsIGVudmlyb25tZW50Lg==[Qq]
[c]IEVudGVyIHdvcmQ=[Qq]
[c]ICo=[Qq]
[f]IE5vLiBIZXJlJiM4MjE3O3MgYSBoaW50LiBFbmRvdGhlcm1zIGdlbmVyYXRlIHRoZWlyIGhlYXQgaW50ZXJuYWxseSwgYW5kIHRoZWlyIGJvZHkgdGVtcGVyYXR1cmUgcmVtYWlucyBjb25zdGFudCBkZXNwaXRlIGNoYW5nZXMgaW4gdGhlIGV4dGVybmFsIGVudmlyb25tZW50Lg==[Qq]
[q]When it comes to body temperature, almost all birds and mammals generate their body heat internally, making them [hangman].
[c]ZW5kb3RoZXJtcw==[Qq]
[q]In the diagram below, you can see how the body temperature of the snake rises and falls with the temperature of the environment. That makes the snake a [hangman] for body temperature.
[c]Y29uZm9ybWVy[Qq]
[q] The body temperature of the wolf shown at “B” stays constant, despite changes in the environment. The general term for the type of homeostatic regulation shown by the wolf would be [hangman]
[c]cmVndWxhdG9y[Qq]
[q][hangman] is a more accurate way to say “cold-blooded,” and [hangman] is more accurate than “warm-blooded.”
[c]RWN0b3RoZXJtaWM=[Qq]
[c]ZW5kb3RoZXJtaWM=[Qq]
[q]The optimal level around which a physiological variable fluctuates is called a [hangman] [hangman]
[c]c2V0[Qq]
[c]cG9pbnQ=[Qq]
[x][restart]
[/qwiz]
4. Adaptations for Regulating Body Temperature
Let’s look at some general mechanisms by which organisms can control their body temperature. Most of these apply to endotherms like mammals and birds, and function to minimize the amount of energy they need to do to keep their body temperature constant.
a. Insulation
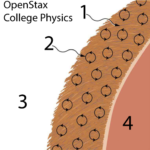
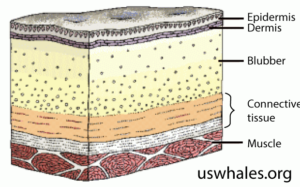
Mammals and birds that live in cold environments use fur (1 at left) or feathers to trap a layer of air (2) next to their bodies (4). Blubber, a thick layer of insulating fat, is another type of insulation that’s particularly important for marine mammals like whales and walruses.
Note that insulation doesn’t have to be an all-or-nothing kind of adaptation. If you have a dog that sheds its coat in the winter, then you’re aware that fur-based insulation can be seasonal. Mammals also have tiny muscles attached to their hair follicles that are called arrector pili. Contraction of these muscles causes hair or fur to stand on end. This increases the amount of air that hair or fur can trap. When you feel goosebumps from the cold you’re experiencing an evolutionary vestige of this adaptation: we humans still have the arrector pili muscles, but we’ve lost most of the insulating body hair.
b. Evaporative cooling
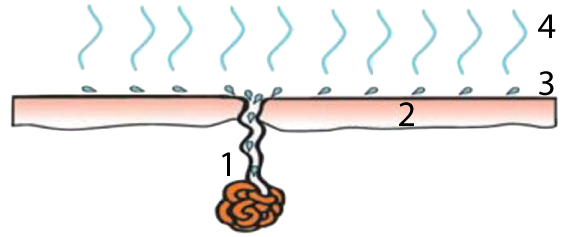
When water molecules evaporate from the skin (or other surfaces), they carry heat energy away from the body, lowering body temperature. This is called evaporative cooling: cooling that happens through evaporation. In humans, evaporative cooling occurs through sweating. Sweat glands (1) release sweat (3) onto the skin (2). Number 4 indicates evaporation.
In dogs and other animals, evaporative cooling occurs through panting. If you’ve seen a video of elephants squirting water onto their backs on a hot day, that’s another way to bring about evaporative cooling.
C. Vasodilation and Vasoconstriction
Vasodilation and vasoconstriction can be used to promote and prevent heat loss. In vasodilation (1) the tiny muscles around the blood vessels that supply the skin and other extremities with blood relax. This allows blood to get closer to the body’s outer surface, which in turn allows heat (2) to radiate from the blood, through the skin (3), and out into the environment. This is also called radiative cooling. When you’re warm and your skin feels flushed, that’s vasodilation. In vasoconstriction (4), the blood vessels supplying the skin and extremities constrict. This keeps blood from flowing close to the skin, decreasing the amount of heat that can flow from the blood out to the body (notice the smaller arrow at “5”). The key thing to remember is that vasodilation increases radiative cooling, while vasoconstriction decreases it.
D. Countercurrent exchange to minimize heat loss
Countercurrent exchange systems are anatomical adaptations that keep heat in the body’s core. Extremities like the feet are allowed to drop in temperature, and circulating blood is organized in a way that minimizes heat loss to the environment.
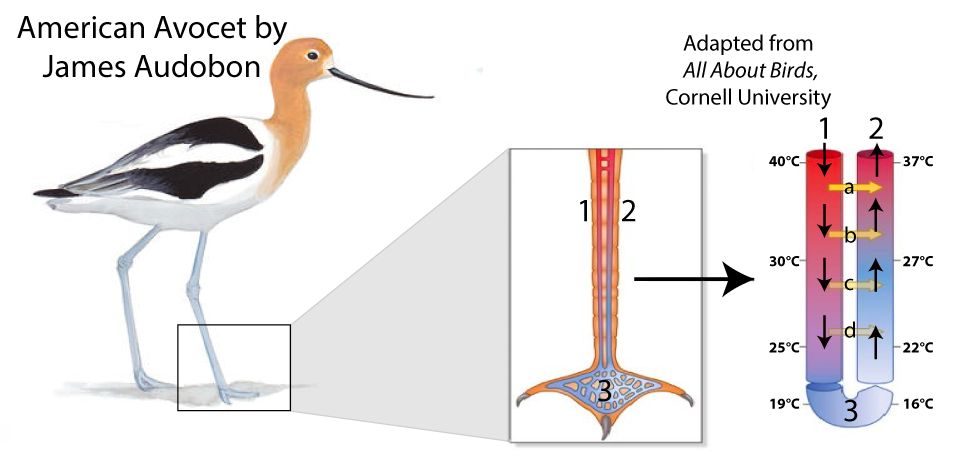
Consider the American Avocet, a shorebird that spends its days wading in along the shores of, among other places, the San Francisco Bay. The water temperature might be 15°C, while the bird’s body temperature runs about 40°C (a bit warmer than us mammals).
If the blood that the avocet was circulating to its legs came back into the bird’s body at 15°C, it would take a lot of energy to warm that blood back up to 40°C. The avocet avoids that energy expenditure through two adaptations. First, the temperature in the legs is allowed to drop significantly below the bird’s core body temperature, with no ill effect on the bird’s overall health. That shouldn’t sound too extraordinary to you, because humans do the same thing: the temperature of our feet is often significantly below 37°C (our core body temperature).
Secondly, outgoing arteries, with warm blood from the body’s core, are interior to and pressed right up against the more exterior veins, which bring blood back to the body. With this arrangement, there’s always a slight temperature gradient. Heat will leave the artery (shown at “1”) and diffuse into the vein (at “2”). At the foot (“3”), the blood will be quite cold (nearly the temperature of the environment). But by the time the blood is ready to reenter the core (at “a”) it has warmed up just below the bird’s body temperature, and very little energy needs to be spent to bring it up to 40°C.
E. Adjusting Surface Area
On an evolutionary scale, animals have evolved body surfaces in response to thermoregulatory demands. Animals in hot environments often have large flaps of skin that act as heat radiators. Examples include the ears of elephants or jackrabbits.
The size of whales can also be explained as an adaptation for thermoregulation. As the ancestors of whales colonized the seas over the past 50 million years, a selective factor guiding their evolution was the energy required to maintain their mammalian body temperature while in cool ocean water. In this context, any ancestral mammal that was too small would be at a disadvantage. Why? Because small animals have a lot of surface area relative to their volume. A small body would radiate a lot of heat into the environment, requiring lots of energy to keep the core temperature at the mammalian set point of 37C.
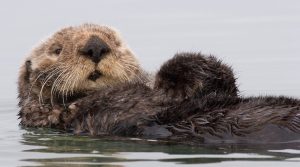
As a result, there’s a minimum body size for marine mammals: none are smaller than sea otters. At the same time, as far as thermoregulation goes, bigger is better. The larger an animal’s body, the smaller its ratio of surface area to volume. So it’s no wonder that whales evolved to be the largest animals on earth. Just by evolving to be big, they’ve reduced the relative amount of energy they need to keep their bodies warm. You can review all of this in our tutorial about surface area and volume.
F. Thermoregulatory Behaviors
In addition to the structural adaptations and physiological responses listed above, there are also a host of behaviors that animals use to thermoregulate. When ectothermic lizards are basking in the sun, they’re raising their body temperature. When they’re too hot, they move into the shade. We mammals, despite our ectothermic metabolisms, do the same.
5. Checking Understanding: Adaptations for thermoregulation
[qwiz qrecord_id=”sciencemusicvideosMeister1961-Adaptations for Thermoregulation (v2.0)”]
[h]Checking Understanding: Adaptations for Thermoregulation
[i]
[q] The diagram below shows how mammals can use fur (“1”) to trap a layer of [hangman] that insulates their bodies (“4”) from the colder environment. This adaptation reduces the amount of [hangman] required to keep their bodies warm.
[c]IGFpcg==[Qq]
[f]IEV4Y2VsbGVudCE=[Qq]
[c]IGVuZXJneQ==[Qq]
[f]IEdyZWF0IQ==[Qq]
[q] In the diagram below, the question mark shows how a thick layer of [hangman] can provide insulation in animals like marine mammals (particularly whales).
[c]IGJsdWJiZXI=[Qq]
[f]IEV4Y2VsbGVudCE=[Qq]
[q] The diagram below shows how [hangman] (at “3”) can reduce body temperature through a process called [hangman]
cooling.
[c]IHN3ZWF0[Qq]
[f]IEdvb2Qh[Qq]
[c]IGV2YXBvcmF0aXZl[Qq]
[f]IEdvb2Qh[Qq]
[q] The diagram below contrasts [hangman] shown at number “1,” with [hangman], shown at “2.”
[c]IHZhc29kaWxhdGlvbg==[Qq]
[f]IEdyZWF0IQ==[Qq]
[c]IHZhc29jb25zdHJpY3Rpb24=[Qq]
[f]IEdvb2Qh[Qq]
[q] Vasodilation [hangman] the amount of [hangman]that an organism can radiate into the environment by allowing more [hangman] to reach the tissues just underneath the outer layers of the [hangman].
[c]IGluY3JlYXNlcw==[Qq]
[f]IEdvb2Qh[Qq]
[c]IGhlYXQ=[Qq]
[f]IEdvb2Qh[Qq]
[c]IGJsb29k[Qq]
[f]IENvcnJlY3Qh[Qq]
[c]IHNraW4=[Qq]
[f]IEdvb2Qh[Qq]
[q] The diagram below illustrates how in a(n) [hangman] exchange system, outgoing blood in an [hangman] (at “1”) exchanges heat with incoming blood in a [hangman]. As a result, the temperature in extremities can drop way[hangman]the temperature in the body’s core, but the temperature of the blood entering the core is never more than a few degrees less than body temperature, which preserves a lot of[hangman].
[c]IGNvdW50ZXJjdXJyZW50[Qq]
[f]IENvcnJlY3Qh[Qq]
[c]IGFydGVyeQ==[Qq]
[f]IEdyZWF0IQ==[Qq]
[c]IHZlaW4=[Qq]
[f]IENvcnJlY3Qh[Qq]
[c]IGJlbG93[Qq]
[f]IEdyZWF0IQ==[Qq]
[c]IGVuZXJneQ==[Qq]
[f]IENvcnJlY3Qh[Qq]
[q] The ears of the jackrabbit shown below are an adaptation for increasing body [hangman] [hangman]. This allows heat to more easily diffuse from the rabbit’s [hangman] into its environment.
[c]IHN1cmZhY2U=[Qq]
[f]IEdvb2Qh[Qq]
[c]IGFyZWE=[Qq]
[f]IENvcnJlY3Qh[Qq]
[c]IGJsb29k[Qq]
[f]IENvcnJlY3Qh[Qq]
[q]Mammals use thermoregulation to keep their body temperature at about 37 C. That means that 37 C is the mammalian temperature [hangman] [hangman].
[c]c2V0[Qq]
[c]cG9pbnQ=[Qq]
[/qwiz]
What’s next?
- Please continue to Topic 4.5, Part 2: Feedback Loops (the next tutorial in AP Bio Topic 4.5)