1. What is Cellular Compartmentalization?
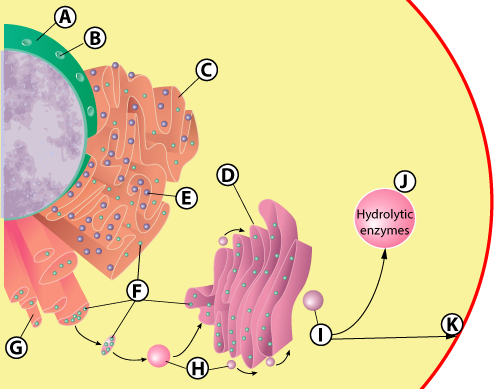
The Endomembrane System, discussed in the previous tutorial, is a great example of an important biological phenomenon: cellular compartmentalization.
A compartment is a separate space within a larger whole, such as the luggage compartment that’s underneath a bus or an airplane. Cellular compartmentalization is a kind of cellular organization that divides the internal volume of the cell into discrete (but often interconnected) compartments. These compartments each have distinct properties and functions.
The cell’s endomembrane system is all about compartmentalization. As we’ve seen, it allows cells to organize processes with assembly-line efficiency. Consider a protein that’s designed for export from the cell. It’s first synthesized in the rough ER (C), then packaged into a vesicle (H) for transport to the Golgi apparatus, then modified by enzymes in the Golgi (D), then repackaged into a vesicle (I) for export from the cell.
In addition to the endomembrane components discussed above, the nucleus, lysosomes, and transport vesicles are also part of the endomembrane system. But these are not the only compartments found in cells. Additional cellular compartments include:
- mitochondria (found in all eukaryotic cells)
- chloroplasts (found in plants and algae)
- vacuoles (found in all eukaryotic cells)
- the large central vacuole (found in plants)
- storage plastids (found in plants, such as the ones that store starch in a potato cell)
Despite the advantages of compartmentalization, it’s not a universal feature of life. To see why that is, we need to look at life’s three domains, their relationship to one another, and how they’ve evolved and diverged over time.
2. Cellular Compartmentalization is Widespread only in Domain Eukarya
Compartmentalization of cellular functions into membrane-bound organelles is widespread in only one of life’s three domains: Eukarya. A domain is the most inclusive group used to classify organisms. The three domains — Eukarya, Bacteria, and Archaea — differ from one another in fundamental ways that relate to cell structure, DNA organization, and metabolism.
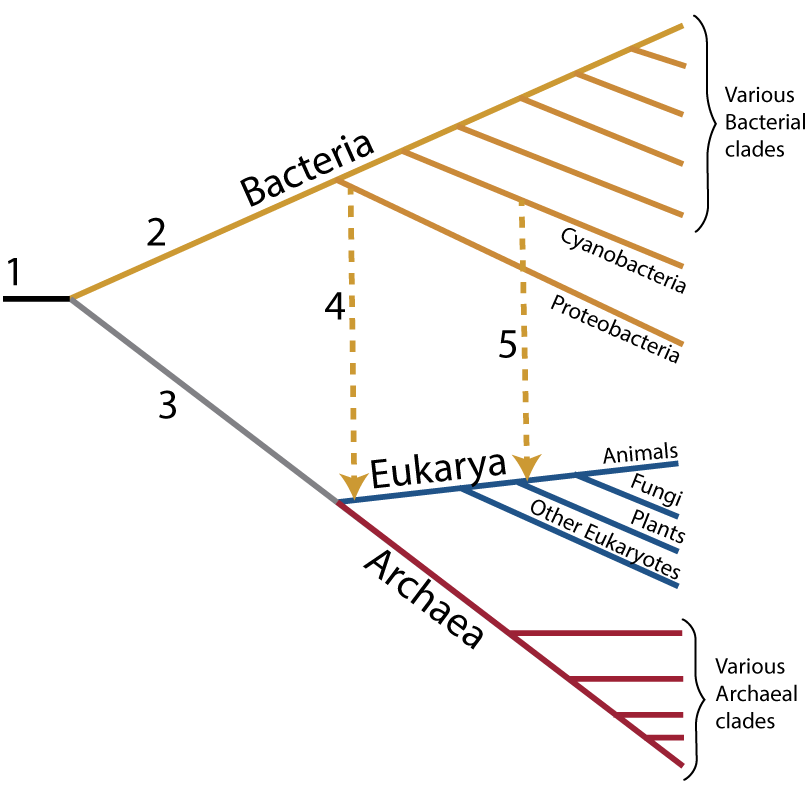
For a very high-level look at the three domains, examine the phylogenetic tree on your right. A phylogenetic tree represents evolutionary relationships. The basic idea of this phylogenetic tree is that all life emerged from a common ancestor (at “1”), which then diverged into the three domains of life. (Note: the term clade which is used in the phylogenetic tree means “a group with a common ancestor”).
Bacteria are on the upper branch of the tree. Bacteria include the E. coli bacteria that live in our guts, the photosynthetic cyanobacteria that live in oceans and freshwater, and hundreds of bacterial species that act as decomposers in the soil.
From a metabolic standpoint, bacteria are phenomenally diverse. Some get energy by stealing electrons from metal. Others use sulfur for energy-related processes. But in terms of structure, all bacteria are small, unicellular, and prokaryotic: no nucleus, no specialized organelles, and very little compartmentalization.
The Archaea are in red on the bottom of the tree. Superficially, Archaea are a lot like bacteria. Their cells are also small and prokaryotic. They’re all unicellular. But a deeper look reveals that Archaea and Bacteria are extremely distant cousins. Here’s the evidence supporting that claim.
- Most Archaea use proteins called histones to package their DNA. Bacteria don’t.
- All Archaea share common ribosomal RNA sequences. These common sequences, discovered by Carl Woese in the 1970s, were the original evidence indicating that all Archaea share a common ancestor and constitute their own domain.
- Archaea don’t use phospholipids to build their cell membranes (as do Eukaryotes and Bacteria). Instead, they use a different lipid (a glycerol-ether lipid).
To give this some context, an archaean and a bacterium are less related to one another than we humans are to an orchid, or orchids are to bread mold.
The Eukarya, shown in blue, is our group. It includes animals, plants, fungi, and a variety of unicellular eukaryotic groups. We eukaryotes have complex, compartmentalized cells. These cells possess mitochondria. Our DNA is organized into multiple chromosomes, and housed in a nucleus that’s separated from the cytoplasm by a nuclear membrane. Eukaryotic cells are much larger than prokaryotic cells, ranging from 10 to 100 micrometers in size. And only eukaryotes have progressed to multicellularity. When you look around at living things, the plants, animals, and fungi that you’re looking at are all eukaryotes.
Now that we understand the three domains, we can address the key question: why is cellular compartmentalization only widespread among eukaryotes? One astonishing answer: it’s because eukaryotes arose through a kind of cellular fusion that involved the other two domains.
We’ll explain this, and present two models of how it might have happened, below. But first, some review.
3. Flashcards: Cellular compartmentalization and the three Domains
[qdeck bold_text=”false” qrecord_id=”sciencemusicvideosMeister1961-Cellular Compartmentalization FC (2.0)”]
[h]Cellular Compartmentalization and the Three Domains
[q]What is cellular compartmentalization?
[a]Cellular compartmentalization is a kind of cellular organization that divides the internal volume of the cell into discrete (but often interconnected) compartments. These compartments each have distinct properties and functions.
[q]List some examples of cellular compartmentalization.
[a]Examples of cellular compartmentalization include the entire endomembrane system (nucleus, rough and smooth E.R., Golgi, lysosomes, transport vesicles), small vacuoles, the large central vacuole in plant cells, mitochondria, chloroplasts, and plastids).
[q]List life’s three domains
[a]The three domains are Bacteria, Archaea, and Eukarya.
[q]Compare and contrast Bacteria and Archaea.
[a]Both Bacteria and Archaea consist of unicellular organisms with small prokaryotic cells. However, a deeper look at DNA organization, ribosomal RNA, and cell membrane structure shows that the two domains are only distantly related.
[q]What are the key features of Domain Eukarya?
[a]The Eukara are eukaryotes. They have complex, compartmentalized cells. These cells possess mitochondria. Their DNA is organized into multiple, linear chromosomes, and housed in a nucleus that’s separated from the cytoplasm by a nuclear membrane. Eukaryotic cells are much larger than prokaryotic cells, ranging from 10 to 100 micrometers in size. And only eukaryotes have progressed to multicellularity.
[q]In terms of cellular compartmentalization, how do the three domains differ?
[a]Cellular compartmentalization is only widespread in Domain Eukarya.
[/qdeck]
4. Eukaryotes arose through Endosymbiosis
Endosymbiosis is a biological relationship in which one species lives inside another. Many forms of endosymbiosis are negative. They involve a parasite living inside of and harming a larger host. Parasitic tapeworms (more common in dogs than people, but common in both) are endosymbiotic parasites. If you want to learn about their lifecycle, follow the preceding link.
But endosymbiosis can also be mutualistic: a win-win relationship that benefits both sides.
|
|
The giant green sea anemone, shown above, is an example of mutualistic endosymbiosis. This animal lives along the rocky shores of the Pacific Coast of North America. It’s primarily a predator, using its tentacles to sting, capture and engulf prey like snails, crabs, and fish. But giant green anemones also have a mutualistic endosymbiont: an alga that lives inside its tissues, and which gives the anemone its green color. The algae perform photosynthesis, secreting excess glucose into the tissues of the anemone, which uses the glucose for cellular respiration. The algae, in return, get a secure habitat. Both the algae and the anemone benefit.
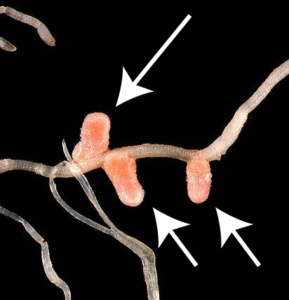
Here’s another example. The plants in the pea family (also known as legumes) acquire nitrogen through a mutualistic relationship they have with various nitrogen-fixing bacteria that they host in specialized nodules in their roots. The plants get nitrogen, an essential element for building proteins and DNA. In return, the bacteria get food from the plant.
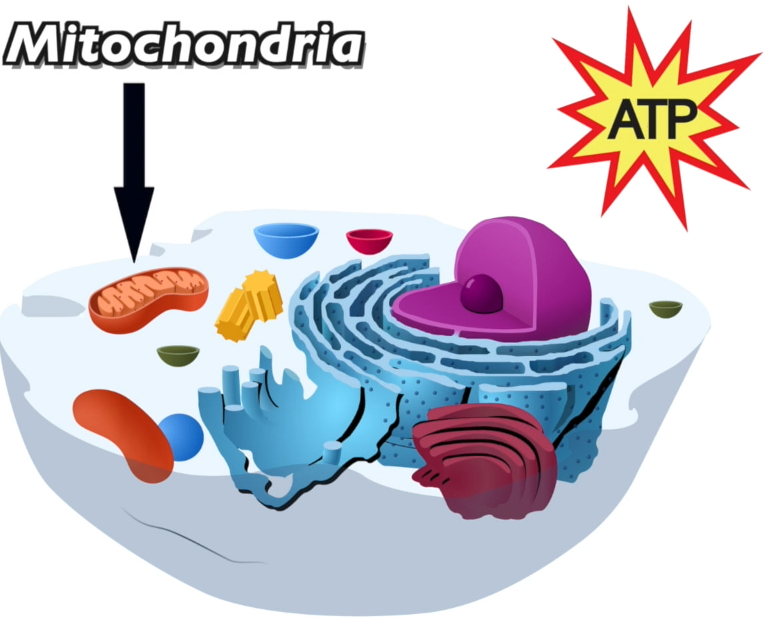
What’s the connection between mutualistic endosymbiosis and the eukaryotes? Astonishingly, each one of your cells is a host to up to 10,000 mutualistic endosymbionts. These endosymbionts are your mitochondria, each one of which is essentially a bacterial cell in its own right, possessing its own genes and ribosomes, and replicating on its own. The relationship is mutualistic because the mitochondria receive food and oxygen from their host cell, and, in return, the mitochondria provide their host (your cells) with ATP, the molecule cells use to perform the work of staying alive.
This partnership is ancient. It’s been going on for two billion years. Over that period, the relationship has become mutually obligatory. Mitochondria are completely dependent upon their host cells to survive. Unlike their free-living bacterial cousins, mitochondria can’t survive independently outside of their host cells: you’ll never find mitochondria growing in the soil, or see them cultured on a petri dish. And our cells are just as dependent for their survival upon their mitochondria. That’s because up to 90% of the ATP that a eukaryotic cell uses is generated by its mitochondria.
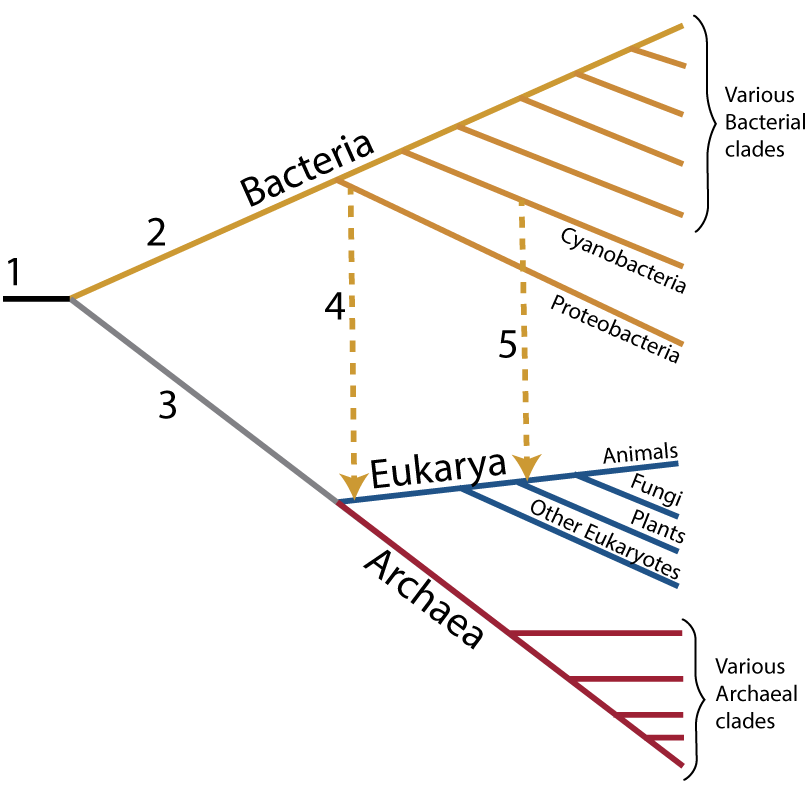
The details of how this endosymbiosis first evolved are the subject of continuing debate. The general story was most forcefully articulated by evolutionary biologist Lynn Margulis in the 1960s and 70s (the link takes you to her Wikipedia page).
You can follow what happened on the phylogenetic tree on the left. Note that time is an implied horizontal axis, with the left side as the past and the right side as the present.
“1” is the common ancestor of all living things, which emerged about 3.8 billion years ago. Almost immediately, life split into two branches: the Bacteria and the Archaea.
About two billion years ago, an archaeal cell took up a bacterial cell. We’ll discuss how that happened below. This is represented on the left by the dotted arrow at “4.” All eukaryotes resulted from this acquisition, and the bacterial cell that was taken up evolved, over time, into the mitochondria that are found in all eukaryotic cells.
Later, a eukaryotic cell (one already possessing mitochondria) took up a cyanobacterium (a photosynthetic bacterium). This acquisition is shown by the dotted arrow at “5,” and it produced the lineage leading to plants and algae. In this case, the cyanobacterial ancestor, over billions of years, evolved into the chloroplast.
Here’s a summary of the evidence that mitochondria and chloroplasts evolved from free-living bacterial cells that were taken up by another cell.
- Both mitochondria and chloroplasts have a double membrane. The outer membrane is a vestige of the membrane of the archaeal cell that engulfed the bacterial cells that later evolved into mitochondria or chloroplasts.
- Uniquely among the cell’s organelles, mitochondria and chloroplasts have their own DNA, and this DNA has the same form (a looped chromosome) as the DNA that’s found in bacteria.
- Both mitochondria and chloroplasts have their own ribosomes and produce some of their own proteins (though many mitochondrial and chloroplast genes have been transferred to the host cell, which also produces many mitochondrial and chloroplast proteins).
- Both mitochondria and chloroplasts replicate themselves autonomously (independently of the host cell’s cell cycle).
All of this evidence points to the same conclusion: that mitochondria and chloroplasts are themselves cells. They just happen to be cells that live inside of other cells.
And how do we know that the original host was an archaean? This idea is mostly based on molecular genetic similarities between eukaryotes and Archaeans. In both domains, the cells
- Package up their DNA by wrapping it around proteins called histones.
- Replicate their DNA using similar mechanisms.
How does that story of cellular fusion explain cellular compartmentalization? We’ll see below that there are at least two very different models for how cellular compartmentalization arose. But first, let’s consolidate what’s above with a few more flashcards.
5. Flashcards: Endosymbiosis and Eukaryotes
[qdeck bold_text=”false” qrecord_id=”sciencemusicvideosMeister1961-Endosymbiosis and Eukaryotes FC (2.0)”]
[h]Endosymbiosis and Eukaryotes
[q]Define endosymbiosis.
[a]Endosymbiosis is a biological relationship in which one species lives inside another.
[q]Compare and contrast negative and positive endosymbiosis.
[a]In negative symbiosis, a parasitic endosymbiont lives inside a larger host, causing that host harm. In positive endosymbiosis, the endosymbiont benefits, and the host benefits. This win-win relationship is called mutualism.
[q]Define mutualism, and briefly describe two examples of mutualistic endosymbiosis.
[a]Mutualism is a relationship between two species in which both benefit. It’s a win-win relationship. Two examples of endosymbiotic mutualism are
- The relationship between the giant green sea anemone and the algae that live within its tissues. The anemones receive sugar produced by the algae through photosynthesis. The algae get a safe place to live
- The relationship between legumes (plants in the pea family) and the nitrogen-fixing bacteria they harbor in their roots. The legumes get nitrogen in a form they can use to build their proteins and nucleic acids. The nitrogen-fixing bacteria get food from the roots and a safe place to live.
[q]Which two eukaryotic organelles are endosymbiotic mutualists?
[a]The two endosymbiotic mutualists in eukaryotic cells are mitochondria (found in all eukaryotic cells) and chloroplasts (found in plants, algae, and other aquatic photosynthetic single-celled eukaryotes).
[q]Describe the mutualistic relationship between eukaryotic cells, mitochondria, and chloroplasts.
[a]All eukaryotic cells benefit from their relationship with mitochondria because they receive ATP (the molecule that cells use to perform any kind of cellular work) from their mitochondria. The mitochondria benefit because they receive food (in the form of pyruvic acid, a by-product of glucose breakdown) from their host cells, as well as a safe place to live.
Plant and algae cells benefit from their relationship with chloroplasts because the chloroplasts perform photosynthesis, enabling them to provide their host cells with food in the form of secreted glucose. The chloroplasts get a safe place to live.
[q]What’s happening at the dotted lines shown at 4 and 5 in the phylogenetic tree?
[a]Arrow “4” indicates the uptake of an ancestral mitochondrion into an archaeal cell. That led to the endosymbiotic merger that gave birth to the eukaryotes.
Arrow “5” indicates the uptake of an ancestral chloroplast into a eukaryotic cell. This endosymbiotic merger gave rise to the plants (and a few related groups of algae not shown in the phylogenetic tree).
[q]What’s the evidence supporting the claim that mitochondria and chloroplasts were once independent cells?
[a]
- Both mitochondria and chloroplasts have a double membrane.
- Mitochondria and chloroplasts have their own DNA, and this DNA has the same form (a looped chromosome) as the DNA that’s found in bacteria.
- Both mitochondria and chloroplasts have their own ribosomes and produce some of their own proteins.
- Both mitochondria and chloroplasts replicate themselves autonomously (independently of the host cell’s cell cycle).
[/qdeck]
6. Two Competing Models for the Evolution of Endosymbiosis and Compartmentalization
The endosymbiotic merger of an ancestral mitochondrion with an archaeal cell was one of the key moments in the evolution of life. It produced our domain: the eukaryotes. As explained by biochemist Nick Lane, possessing multiple ATP-secreting mitochondria made much more ATP available/cell. This enabled eukaryotic cells to grow in size and complexity. That made the transition from unicellularity to multicellularity possible. Multicellularity, in turn, made us possible.
But how did this endosymbiotic merger happen? And did endosymbiosis precede compartmentalization, or result from it?
In what’s below, you’ll look at two competing models that explain the origin of eukaryotic cells.
6a.Model 1: A compartmentalized proto-eukaryote engulfs a bacterium
In many textbooks (Campbell, Biology by Pearson; Principles of Life by Sinauer), the story of endosymbiosis and the origins of eukaryotic compartmentalization runs as follows.
Step 1: A prokaryotic cell (1) lost its cell wall, leaving it with a membrane (b) and genetic material (a).
Step 2: Infoldings of the membrane (not shown) caused the cell (shown at 2) to develop an internal membrane system, with an endoplasmic reticulum (d) and vacuoles (e).
Step 3: Without a wall, this cell (3) could now adopt a phagocytic lifestyle, engulfing other cells (such as the one at “f”)
Step 4: At some point, one engulfed bacterial cell (at “f”) was not digested for food, but managed to stay alive inside its host. This engulfed bacterium developed into an ATP-secreting mitochondrion (at “g”). This type of cell (at 4) became the ancestor of all eukaryotes.
Step 5: Later, a eukaryotic cell (one already possessing mitochondria, such as the one shown at “5”), engulfed a cyanobacterium (at “h”). Cyanobacteria are bacterial cells that perform photosynthesis.
Step 6: This engulfed cell evolved, over time, into the chloroplasts (at “j”) found in plants and algae.
To summarize: the basic idea behind this scenario is that eukaryotic compartmentalization evolved first (steps 1 and 2 above), and then endosymbiosis took place (steps 3 and 4 for all eukaryotes, steps 5 and 6 as an additional step for plants and algae).
6b. Model 2: Endosymbiosis led to compartmentalization
While Model 1 is widely presented in textbooks, it has several problems.
Evolution has no foresight. What was the evolutionary pressure that would have led the prokaryotic cell at number 1 to make the energy-intensive changes in steps 2 and 3?
- Phagocytosis is a uniquely eukaryotic activity. Amoebas do it. Our white blood cells do it as part of the immune response. But no prokaryotic cells are phagocytic. Why would phagocytosis evolve in one prokaryotic line, and then disappear? In addition, phagocytosis requires quickly extending and changing the shape of the cell membrane, which requires a lot of energy. That kind of energy is available to eukaryotic cells because they possess mitochondria, which secrete ATP. How could that amount of energy be available in cells without mitochondria?
Partly in response to these criticisms, Model 2 was developed. In this model, endosymbiosis occurred first, and compartmentalization followed.
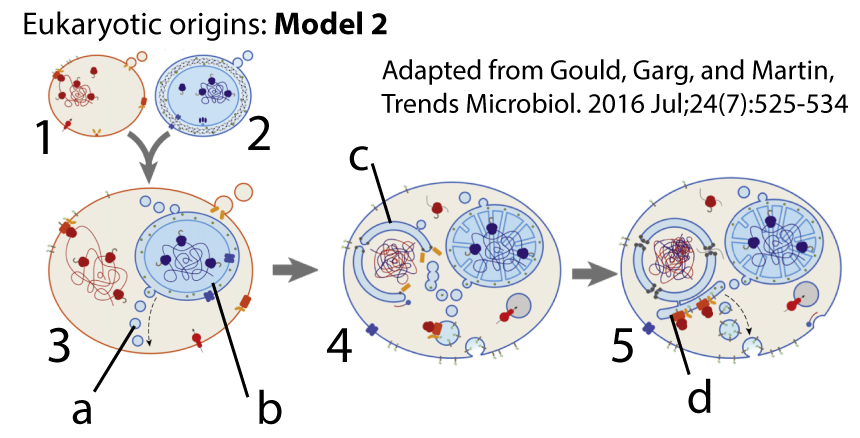
In this model, a bacterial cell (shown at 2) entered into an archaeal cell (at 1). Once inside, the bacterial cell (now a proto-mitochondrion, at “b”) secreted vesicles (shown at “a”) which accumulated inside its new host (image “3”). In image 4, these secreted vesicles become the source for the nuclear membrane (at “c”). That nuclear membrane protects the genetic material of the host cell. Later, in image 5, additional vesicles produce the endoplasmic reticulum (shown at “d”) and, by extension, the other organelles of the endomembrane system.
When the proto-mitochondrion (“b”) reproduces, the host cell now has even more ATP available. At the same time, there’s also evolutionary pressure for the host cell to grow bigger (to make room for its growing population of mitochondria) and to acquire more food (to feed its mitochondria). This sets the stage for this newly emerging eukaryotic cell to grow in size and ultimately evolve into new lifestyles, such as phagocytosis.
Model 2 was developed by biologists like William Martin and popularized by biochemist Nick Lane. To me, it seems more plausible than model 1. I’ve put links to additional articles about the origins of eukaryotes below. I suggest you learn both models (and both diagrams are in the quiz below).
7. Checking Understanding: Eukaryotic origins and Cellular compartmentalization
Are your mitochondria yours? Are they organelles that do your bidding? Or are you doing their bidding?
Here’s how writer/physician Lewis Thomas explained it in his book “The Lives of a Cell:”
We are shared, rented, occupied. At the interior of our cells, driving them, providing the oxidative energy that sends us out for the improvement of each shining day, are the mitochondria, and in a strict sense, they are not ours.
It’s something to think about!
Let’s see how well you’ve mastered this material.
[qwiz qrecord_id=”sciencemusicvideosMeister1961-Eukaryotic Origins and Compartmentalization Quiz”] [h]
Cellular Compartmentalization: Checking Understanding
[i]Mitochondria
Making ATP
Setting the stage for
Life’s complexity
[q] Organelles like the mitochondria, the Golgi, and lysosomes are all examples of cellular [hangman].
[c]IGNvbXBhcnRtZW50YWxpemF0aW9u[Qq]
[f]IENvcnJlY3Qh[Qq]
[q] The only domain in which extensive cellular compartmentalization is found is domain [hangman].
[c]ZXVrYXJ5YQ==[Qq]
[f]IEV4Y2VsbGVudCE=[Qq]
[q] In the diagram below, which arrow shows the acquisition of a bacterial cell by an archaeal cell that led to mitochondria.
[textentry single_char=”true”]
[c]ID Q=[Qq]
[f]IEV4Y2VsbGVudC4gJiM4MjIwOzQmIzgyMjE7IHNob3dzIHRoZSBhY3F1aXNpdGlvbiBvZiBhIGJhY3RlcmlhbCBjZWxsIHRoYXQsIG92ZXIgdGltZSwgZXZvbHZlZCBpbnRvIHRoZSBtaXRvY2hvbmRyaWEu[Qq]
[c]IEVudGVyIHdvcmQ=[Qq]
[c]ICo=[Qq]
[f]IE5vLiBIZXJlJiM4MjE3O3MgYSBoaW50LiBNaXRvY2hvbmRyaWEgYXJlIGZvdW5kIGluIGFsbCBldWthcnlvdGljIGNlbGxzLiBXaGljaCBhcnJvdyBpcyBwb2ludGluZyB0byB0aGUgYmFzZSBvZiB0aGUgZXVrYXJ5b3RpYyBicmFuY2ggb2YgdGhlIHRyZWUgb2YgbGlmZT8=[Qq]
[q] In the diagram below, which arrow shows the acquisition of a bacterial cell by a eukaryotic cell that led to chloroplasts.
[textentry single_char=”true”]
[c]ID U=[Qq]
[f]IE5pY2UuICYjODIyMDs1JiM4MjIxOyBzaG93cyB0aGUgYWNxdWlzaXRpb24gb2YgYSBiYWN0ZXJpYWwgY2VsbCB0aGF0LCBvdmVyIHRpbWUsIGV2b2x2ZWQgaW50byB0aGUgY2hsb3JvcGxhc3Qu[Qq]
[c]IEVudGVyIHdvcmQ=[Qq]
[c]ICo=[Qq]
[f]IE5vLiBIZXJlJiM4MjE3O3MgYSBoaW50LiBDaGxvcm9wbGFzdHMgYXJlIGZvdW5kIGluIHBsYW50IGNlbGxzLiBXaGljaCBhcnJvdyBpcyBwb2ludGluZyB0byB0aGUgYmFzZSBvZiB0aGUgcGxhbnQga2luZ2RvbT8=[Qq]
[q]The [hangman] are a prokaryotic domain that are, based on their metabolism and molecular structure, actually quite different from bacteria.
[c]QXJjaGFlYQ==[Qq]
[q]Cells that lack a nucleus or other membrane-bound compartments are described as [hangman]
[c]cHJva2FyeW90aWM=[Qq]
[q]The cell type found in animals, plants, fungi, and many single-celled organisms with large complex cells is described as [hangman].
[c]ZXVrYXJ5b3RpYw==[Qq]
[q]The only domain that includes multicellular organisms is the [hangman].
[c]ZXVrYXJ5YQ==[Qq]
[q]A relationship in which one organism lives inside one another is called [hangman].
[c]ZW5kb3N5bWJpb3Npcw==[Qq]
[q]A symbiotic relationship in which both organisms benefit is called [hangman].
[c]bXV0dWFsaXNt[Qq]
[q]Mitochondria can be thought of as [hangman] endosymbionts living within every [hangman] cell. The mitochondrial supply their hosts with [hangman]. In return, the mitochondria receive [hangman] and oxygen.
[c]bXV0dWFsaXN0aWM=[Qq]
[c]ZXVrYXJ5b3RpYw==[Qq]
[c]QVRQ[Qq]
[c]Zm9vZA==[Qq]
[q]In most theories about the origin of mitochondria, the cell that took up the mitochondria would have belonged to the domain [hangman].
[c]YXJjaGFlYQ==[Qq]
[q]Evidence for the endosymbiotic origins of mitochondria and chloroplasts includes the fact that both mitochondria and chloroplasts have a [hangman] membrane; that both possess their own [hangman], which is organized into a looped [hangman]; and that both can [hangman] by binary fission, just like bacteria.
[c]ZG91Ymxl[Qq]
[c]RE5B[Qq]
[c]Y2hyb21vc29tZQ==[Qq]
[c]cmVwcm9kdWNl[Qq]
[q] In the simplified phylogenetic tree shown below, which number indicates the Domain Bacteria?
[textentry single_char=”true”]
[c]ID E=[Qq]
[f]IEV4Y2VsbGVudC4gJiM4MjIwOzEmIzgyMjE7IGluZGljYXRlcyB0aGUgYmFjdGVyaWEu[Qq]
[c]IEVudGVyIHdvcmQ=[Qq]
[c]ICo=[Qq]
[f]IE5vLiBIZXJlJiM4MjE3O3MgYSBoaW50LiBUaGUgZXVrYXJ5b3RlcyBhbmQgYXJjaGFlYSBhcmUgdGhlIHR3byBtb3N0IGNsb3NlbHkgcmVsYXRlZCBkb21haW5zLiBJZiBldWthcnlvdGVzIGFyZSBhdCAmIzgyMjA7MiwmIzgyMjE7IHdoZXJlIHdvdWxkIHRoZSBiYWN0ZXJpYSBoYXZlIHRvIGJlPw==[Qq]
[q] In the simplified phylogenetic tree shown below, which number or letter indicates the origin of eukaryotes through the uptake of mitochondria?
[textentry single_char=”true”]
[c]IG E=[Qq]
[f]IEV4Y2VsbGVudC4gJiM4MjIwO0EmIzgyMjE7IGluZGljYXRlcyB0aGUgb3JpZ2lucyBvZiBldWthcnlvdGVzIHRocm91Z2ggdGhlIHRha2luZyB1cCBvZiBtaXRvY2hvbmRyaWEu[Qq]
[c]IEVudGVyIHdvcmQ=[Qq]
[c]ICo=[Qq]
[f]IE5vLiBIZXJlJiM4MjE3O3MgYSBoaW50LiBNaXRvY2hvbmRyaWEgYXJlIHRoZSBldm9sdXRpb25hcnkgaW5ub3ZhdGlvbiB0aGF0IGxlZCB0byB0aGUgZXVrYXJ5b3Rlcy4=[Qq]
[q] In the simplified phylogenetic tree shown below, which number or letter indicates the Domain Archaea?
[textentry single_char=”true”]
[c]ID M=[Qq]
[f]IEV4Y2VsbGVudC4gJiM4MjIwOzMmIzgyMjE7IGluZGljYXRlcyB0aGUgQXJjaGFlYS4=[Qq]
[c]IEVudGVyIHdvcmQ=[Qq]
[c]ICo=[Qq]
[f]IE5vLiBIZXJlJiM4MjE3O3MgYSBoaW50LiBUaGUgZXVrYXJ5b3RlcyBhbmQgYXJjaGFlYSBhcmUgdGhlIHR3byBtb3N0IGNsb3NlbHkgcmVsYXRlZCBkb21haW5zLiBBbmltYWxzIGFyZSBldWthcnlvdGVzLiBZb3UgZmlndXJlIG91dCB0aGUgcmVzdC4=[Qq]
[q] In the simplified phylogenetic tree shown below, which number or letter indicates the evolution of chloroplasts?
[textentry single_char=”true”]
[c]IG I=[Qq]
[f]IEV4Y2VsbGVudC4gJiM4MjIwO0ImIzgyMjE7IGluZGljYXRlcyB0aGUgYWNxdWlzaXRpb24gb2YgY2hsb3JvcGxhc3RzLg==[Qq]
[c]IEVudGVyIHdvcmQ=[Qq]
[c]ICo=[Qq]
[f]IE5vLiBIZXJlJiM4MjE3O3MgYSBoaW50LiBUaGUgY2hsb3JvcGxhc3RzIGFyZSB0aGUga2V5IGZlYXR1cmUgdGhhdCBkZWZpbmVzIHRoZSBwbGFudCBraW5nZG9tLg==[Qq]
[q] In the simplified phylogenetic tree shown below, which number or letter indicates the only group with complex, compartmentalized cells?
[textentry single_char=”true”]
[c]ID I=[Qq]
[f]IEV4Y2VsbGVudC4gJiM4MjIwOzImIzgyMjE7IGluZGljYXRlcyB0aGUgZXVrYXJ5b3RlcywgdGhlIG9ubHkgZG9tYWluIHdpdGggY29tcGxleCwgY29tcGFydG1lbnRhbGl6ZWQgY2VsbHMu[Qq]
[c]IEVudGVyIHdvcmQ=[Qq]
[c]ICo=[Qq]
[f]IE5vLiBIZXJlJiM4MjE3O3MgYSBoaW50LiBUaGlzIGlzIHRoZSBvbmx5IGRvbWFpbiB0aGF0JiM4MjE3O3M=IG5vdA==IHByb2thcnlvdGljLg==[Qq]
[q] In the diagram below, which number or letter indicates a mitochondrion in a cell that was ancestral to fungi, animals, and some unicellular eukaryotes (but not plants)?
[textentry single_char=”true”]
[c]IG c=[Qq]
[f]IEV4Y2VsbGVudC4gJiM4MjIwO0cmIzgyMjE7IGluZGljYXRlcyB0aGUgbWl0b2Nob25kcmlvbiBpbiBhIG5vbi1waG90b3N5bnRoZXRpYyBjZWxsLiBUaGlzIGNlbGwgd291bGQgaGF2ZSBiZWVuIHRoZSBhbmNlc3RvciBvZiBmdW5naSwgYW5pbWFscywgYW5kIHRoZSBub24tcGhvdG9zeW50aGV0aWMgdW5pY2VsbHVsYXIgZXVrYXJ5b3RlcyAobGlrZSBwYXJhbWVjaWEgb3IgYW1vZWJhcyk=[Qq]
[c]IEVudGVyIHdvcmQ=[Qq]
[c]ICo=[Qq]
[f]IE5vLiBIZXJlJiM4MjE3O3MgYSBoaW50LiBGaW5kIGFuIGVuZG9zeW1iaW90aWMgb3JnYW5lbGxlIHRoYXQmIzgyMTc7cyBpbiBBTEwgZXVrYXJ5b3RlcywgYnV0IGluIGEgY2VsbCB0aGF0IGRvZXNuJiM4MjE3O3QgaGF2ZSBjaGxvcm9wbGFzdHMu[Qq]
[q] In the diagram below, the free-living bacterial cell that evolved into a mitochondrion is shown at
[textentry single_char=”true”]
[c]IG Y=[Qq]
[f]IEV4Y2VsbGVudC4gJiM4MjIwO0YmIzgyMjE7IGluZGljYXRlcyB0aGUgZnJlZS1saXZpbmcgYmFjdGVyaWFsIGNlbGwgdGhhdCBldm9sdmVkIHRvIGJlY29tZSBhIG1pdG9jaG9uZHJpb24u[Qq]
[c]IEVudGVyIHdvcmQ=[Qq]
[c]ICo=[Qq]
[f]IE5vLiBIZXJlJiM4MjE3O3MgYSBoaW50LiBUaGUgbWl0b2Nob25kcmlvbiBpcyB0aGUgZmlyc3Qgb3JnYW5lbGxlIHRvIGhhdmUgYXJpc2VuIGVuZG9zeW1iaW90aWNhbGx5[Qq]
[q] In the diagram below, the free-living photosynthetic bacterial cell that evolved into a chloroplast is shown at
[textentry single_char=”true”]
[c]IG g=[Qq]
[f]IEV4Y2VsbGVudC4gJiM4MjIwO0gmIzgyMjE7IGluZGljYXRlcyB0aGUgZnJlZS1saXZpbmcgcGhvdG9zeW50aGV0aWMgYmFjdGVyaWFsIGNlbGwgdGhhdCBldm9sdmVkIHRvIGJlY29tZSBhIGNobG9yb3BsYXN0Lg==[Qq]
[c]IEVudGVyIHdvcmQ=[Qq]
[c]ICo=[Qq]
[f]IE5vLiBIZXJlJiM4MjE3O3MgYSBoaW50LiBUaGUgY2hsb3JvcGxhc3QgaXMgdGhlIHNlY29uZCBvcmdhbmVsbGUgdG8gaGF2ZSBhcmlzZW4gdGhyb3VnaCBlbmRvc3ltYmlvc2lzLg==[Qq]
[q] In the diagram below, the endoplasmic reticulum is shown at
[textentry single_char=”true”]
[c]IG Q=[Qq]
[f]IEV4Y2VsbGVudC4gJiM4MjIwO0QmIzgyMjE7IGluZGljYXRlcyB0aGUgZW5kb3BsYXNtaWMgcmV0aWN1bHVt[Qq]
[c]IEVudGVyIHdvcmQ=[Qq]
[c]ICo=[Qq]
[f]IE5vLiBIZXJlJiM4MjE3O3MgYSBoaW50LiBUaGUgZW5kb3BsYXNtaWMgcmV0aWN1bHVtIGlzIGFuIGludGVybmFsIG5ldHdvcmsgb2YgY2hhbm5lbHMsIHN1cnJvdW5kZWQgYnkgbWVtYnJhbmUu[Qq]
[q]In the model of eukaryotic origins shown below, [hangman] (such as that which produced the ER and vacuoles) precedes [hangman].
[c]Y29tcGFydG1lbnRhbGl6YXRpb24=[Qq]
[c]ZW5kb3N5bWJpb3Npcw==[Qq]
[q] In the diagram below, an archaeal cell is shown at
[textentry single_char=”true”]
[c]ID E=[Qq]
[f]IE5pY2Ugam9iLiAmIzgyMjA7MSYjODIyMTsgaW5kaWNhdGVzIHRoZSBhcmNoYWVhbCBjZWxsIHRoYXQgdG9vayB1cCBhIGJhY3RlcmlhbCBjZWxsLCB0aGUgY2VsbHVsYXIgYWNxdWlzaXRpb24gdGhhdCBsZWQgdG8gdGhlIGV1a2FyeW90ZXMu[Qq]
[c]IEVudGVyIHdvcmQ=[Qq]
[c]ICo=[Qq]
[f]IE5vLiBIZXJlJiM4MjE3O3MgYSBoaW50LiBUaGUgYXJjaGFlYWwgY2VsbCB3YXMgdGhlIG9uZSB0aGF0LCBhdCB0aGUgYmVnaW5uaW5nIG9mIHRoZSBldWthcnlvdGljIHN0b3J5LCB0b29rIHVwIGEgYmFjdGVyaWFsIGNlbGwu[Qq]
[q] In the diagram below, vesicles secreted by the primitive mitochondria are shown at
[textentry single_char=”true”]
[c]IG E=[Qq]
[f]QXdlc29tZS4gVGhlIGxldHRlciAmIzgyMjA7YSYjODIyMTsgaW5kaWNhdGVzIHZlc2ljbGVzIGJlaW5nIHJlbGVhc2VkIGJ5IHRoZSBwcmltaXRpdmUgbWl0b2Nob25kcmlhLiBVbHRpbWF0ZWx5LCB0aGVzZSB2ZXNpY2xlcyB3aWxsIHByb3ZpZGUgdGhlIG1lbWJyYW5lIHRoYXQgd2lsbCBmb3JtIHRoZSBudWNsZWFyIG1lbWJyYW5lLCB0aGUgb3JnYW5lbGxlcyBvZiB0aGUgZW5kb21lbWJyYW5lIHN5c3RlbSwgYW5kIHRoZQ==[Qq]
[c]IEVudGVyIHdvcmQ=[Qq]
[c]ICo=[Qq]
[f]IE5vLiBIZXJlJiM4MjE3O3MgYSBoaW50LiBWZXNpY2xlcyBhcmUgZmx1aWQtZmlsbGVkIGJ1YmJsZXMgc3Vycm91bmRlZCBieSBhIG1lbWJyYW5lLg==[Qq]
[q] In the diagram below, the developing nuclear membrane is shown by which number or letter?
[textentry single_char=”true”]
[c]IG M=[Qq]
[f]VGVycmlmaWMuIExldHRlciAmIzgyMjA7QyYjODIyMTsgaW5kaWNhdGVzIHRoZSBkZXZlbG9waW5nIG51Y2xlYXIgbWVtYnJhbmUu[Qq]
[c]IEVudGVyIHdvcmQ=[Qq]
[c]ICo=[Qq]
[f]IE5vLiBIZXJlJiM4MjE3O3MgYSBoaW50LiBUaGUgbnVjbGVhciBtZW1icmFuZSBkZXZlbG9wcyBhcm91bmQgdGhlIGdlbmV0aWMgbWF0ZXJpYWwgdGhhdCB3YXMgb3JpZ2luYWxseSBpbiB0aGUgQXJjaGFlYWwgaG9zdCBjZWxsLg==[Qq]
[q] In the diagram below, the developing endoplasmic reticulum is shown by which number or letter?
[textentry single_char=”true”]
[c]IG Q=[Qq]
[f]VGVycmlmaWMuIExldHRlciAmIzgyMjA7ZCYjODIyMTsgaW5kaWNhdGVzIHRoZSBkZXZlbG9waW5nIGVuZG9wbGFzbWljIHJldGljdWx1bS4=[Qq]
[c]IEVudGVyIHdvcmQ=[Qq]
[c]ICo=[Qq]
[f]IE5vLiBIZXJlJiM4MjE3O3MgYSBoaW50LiBUaGUgRVIgaXMgc2hvd24gY2xvc2UgdG8gdGhlIG51Y2xlYXIgbWVtYnJhbmUu[Qq]
[q]In the model of eukaryotic origins shown below, [hangman] occurred first. The engulfed cell released [hangman] that developed into the nuclear [hangman], the endoplasmic [hangman], and the other organelles of the endomembrane system.
[c]ZW5kb3N5bWJpb3Npcw==[Qq]
[c]dmVzaWNsZXM=[Qq]
[c]bWVtYnJhbmU=[Qq]
[c]cmV0aWN1bHVt[Qq]
[x][restart]
[/qwiz]
8. Compartmentalization: Final Points
Cellular compartmentalization is most widespread in eukaryotes. However, there is some compartmentalization in prokaryotes. For example, when cyanobacteria (and chloroplasts, their descendants) perform photosynthesis, they pump protons into a compartment called a thylakoid space, which is surrounded by a thylakoid membrane. Similarly, mitochondria (which are essentially prokaryotic cells) produce ATP by pumping protons into a compartment called the intermembrane space. You’ll learn about these mini-compartments in chloroplasts and mitochondria in Unit 3.
On the level of entire organisms, compartmentalization is an important organizing principle and a key part of structure and function. Think of how you digest food. It’s all about moving food along a digestive assembly line from one specialized compartment to another.
Each compartment plays a different role in the process: your mouth uses teeth to grind the food; your stomach is specialized to disinfect food by soaking it in hydrochloric acid, as well as to digest proteins by exposing them to acid-optimized protein-digesting enzymes; your intestines switch the pH from acidic to basic, and use a variety of enzymes to completely digest what you’ve eaten. Then, specialized intestinal cells absorb the food into your bloodstream.
If you want to learn more, check out this music video and these interactive activities about the digestive system (but be aware: none of this content is on the AP Bio exam!).
9. What’s next?
- Continue to Topic 2.4: Cell Membrane Structure (the next topic in AP Bio Unit 2)
10. Further Reading
Both of the links below open in new tabs. The first is an article written for the general public by one of today’s best science writers, Carl Zimmer. The second is a peer-reviewed article by some of the best thinkers in the origin-of-life field.
- On the Origin of the Eukaryotes (Carl Zimmer)
- Bacterial Vesicle Secretion and the Evolutionary Origin of the Eukaryotic Endomembrane System