1. Cellular compartmentalization
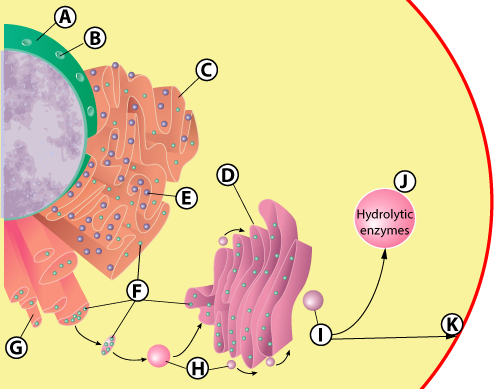
The endomembrane system — the focus of the last tutorial — is an example of cellular compartmentalization. A compartment is a separate space within a larger whole. In relationship to the endomembrane system, the nucleus (A), the rough ER (C), the smooth ER (G), the Golgi apparatus (D), the lysosomes (J), and all the vesicles (H and I) and vacuoles (not shown) are all compartments within the cell, each with distinct properties and functions.
This is also true of organelles that aren’t part of the endomembrane system, such as mitochondria (which are found in all eukaryotic cells) and chloroplasts (found in plants and other photosynthetic eukaryotes, such as algae).
Cellular compartmentalization allows cells to optimize the efficiency of the processes that occur within organelles. But despite the advantages of compartmentalization, it’s not a universal feature of life. In fact, compartmentalization of cellular functions into membrane-bound organelles is limited to only one of life’s three major groups or domains. That domain is the one that we belong to, the Eukarya. In addition to animals, eukarya includes plants, fungi, and other organisms with eukaryotic cells: cells that are relatively large, complex, and compartmentalized. And to see why that is, we need to look at how life has diverged over time.
2. Compartmentalization is present only in Domain Eukarya
The diagram below is very high-level phylogenetic tree. A phylogenetic tree represents evolutionary relationships.
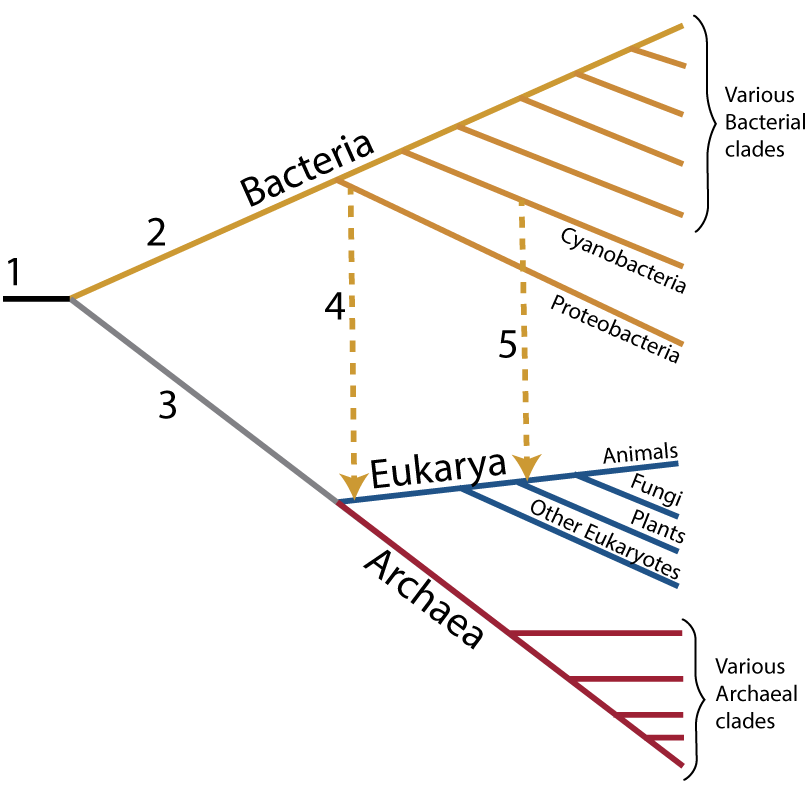
Number 1 represents the common ancestor of all living organisms. see note 1 Shortly after life’s emergence, life split into two major branches. The upper branch (“2”) leads to the Bacteria. Biochemically, bacteria are enormously diverse, but morphologically they’re all small, unicellular, and prokaryotic. That means that 1) their DNA isn’t separated from the rest of the cytoplasm by a nuclear membrane, and 2) they’re not internally compartmentalized (no membrane-bound organelles). The prokaryotic cells that make up bacteria are between 1 to 10 micrometers in diameter (a micrometer is a millionth of a meter)
The lower branch (“3”) leads to life’s two other domains. One of these, indicated in red branches at the bottom, is the Archaea. In terms of form and structure, Archaea are a lot like bacteria. Their cells are also small and prokaryotic. Archaea, in fact, wasn’t recognized as a distinct branch on the tree of life until the 1970s. This recognition came about through the work of Carl Woese, a biologist at the University of Illinois. Woese analyzed the sequences of the RNA that makes up the small subunit of the ribosome (the cell’s protein factory). His analysis revealed that Archaea and Bacteria, despite the fact that they were both prokaryotic in structure, were not an evolutionary unified group. Rather, they’re extremely distant cousins. An archaean and a bacterium are far less related to one another than we humans are to an orchid, or orchids are to bread mold (because humans, orchids, and bread mold are all in the same domain — the Eukarya — the domain that includes all of the eukaryotes).
The Eukarya, shown in blue is our group. We eukaryotes have complex, compartmentalized cells. We possess mitochondria. Our DNA is organized into multiple chromosomes, and housed in a nucleus that’s separated from the cytoplasm by a nuclear membrane. Eukaryotic cells are much larger than prokaryotic cells, ranging from 10 to 100 micrometers in size. And only eukaryotes have progressed to multicellularity. When you look around at living things, the plants, animals, and fungi that you’re looking at are all eukaryotes.
Eukaryotes arose through a kind of cellular fusion that involved the other two domains. The details of how this happened are unclear, but here’s what we know.
3. Eukaryotes arose through Endosymbiosis
Endosymbiosis is a biological relationship in which one species lives inside another. Many forms of endosymbiosis are negative and involve a parasite living inside and harming a larger host (imaging a parasitic tapeworm: that’s an endosymbiotic parasite). But endosymbiosis can also be mutualistic: a win-win relationship that benefits both sides.
|
|
The giant green sea anemone, shown above, is an example of mutualistic endosymbiosis. This animal lives along the rocky shores of the Pacific Coast of North America. It’s primarily a predator, using its tentacles to sting, capture and engulf prey like snails, crabs, and fish. But giant green anemones also have a mutualistic endosymbiont: an alga that lives inside its tissues, and which gives the anemone its green coloration. The algae perform photosynthesis, secreting excess glucose into the tissues of the anemone, which uses the glucose for cellular respiration. The algae, in return, get a secure habitat.
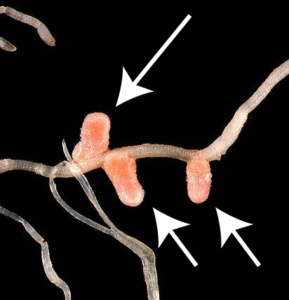
Here’s another example. The plants in the pea family are able to acquire nitrogen because of a mutualistic relationship they have with various nitrogen-fixing bacteria that they host in specialized nodules in their roots. The plants get nitrogen, an essential element for building proteins and DNA. In return, the bacteria get food from the plant.
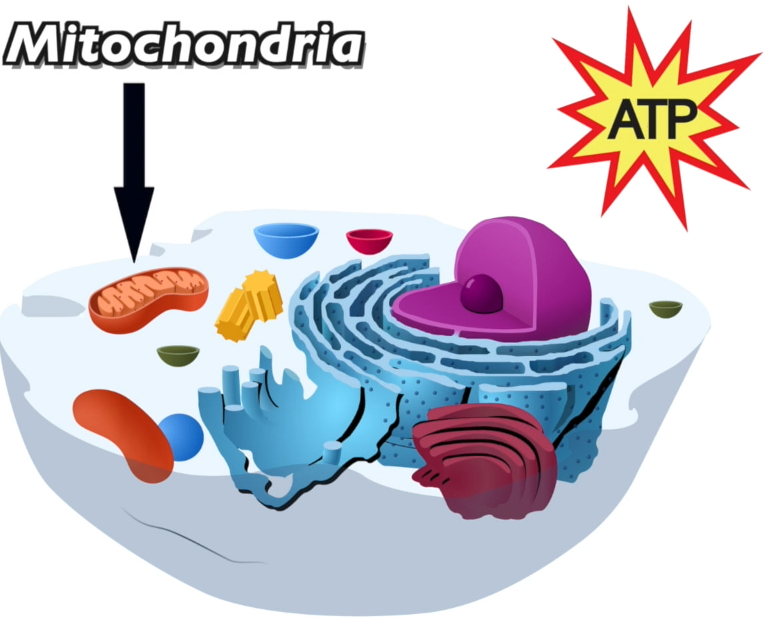
What’s the connection between mutualistic endosymbiosis and the eukaryotes? Astonishingly, each one of your cells is host to up to 10,000 mutualistic endosymbionts. These endosymbionts are the mitochondria, each one of which is essentially a cell in its own right, possessing its own genes and ribosomes, and replicating on its own. The relationship is mutualistic because the mitochondria receive food and oxygen from their host cell, and, in return, the mitochondria provide their host with ATP, the molecule cells use to perform the work of staying alive.
At this point, two billion years after this partnership began, the relationship has become mutually obligatory. Mitochondria are completely dependent upon their host cells in order to survive. Unlike their free-living bacterial cousins, mitochondria can’t survive independently outside of their host cells: you’ll never see mitochondria growing on a petri dish. And our cells are just as dependent for their survival upon their mitochondria since 90% of the ATP that a cell uses is generated by its mitochondria.
The details of how this endosymbiosis occurred are the subject of continuing debate, but here’s the general story, which was most forcefully articulated by evolutionary biologist Lynn Margulis in the 1960s and 70s (the link takes you to her Wikipedia page). About two billion years ago, an archaeal cell took up a bacterial cell. This is represented in Figure 2 above by the arrow at “4.” All the eukaryotes resulted from this acquisition, and the bacterial cell that was taken up evolved, over time, into the mitochondria that are found in all eukaryotic cells. Later, a eukaryotic cell (one already possessing mitochondria) took up a cyanobacterium (a photosynthetic bacterium). This acquisition is shown by the arrow at “5,” and it produced the lineage leading to plants and green algae. In this case, the cyanobacterial ancestor, over billions of years, evolved into the chloroplast.
Here’s a summary of the evidence that mitochondria and chloroplasts evolved from free-living bacterial cells that were taken up by another cell.
- Both mitochondria and chloroplasts have a double membrane. The outer membrane is a vestige of the membrane of the archaeal cell that engulfed the bacterial cells that later became mitochondria or chloroplasts.
- Uniquely among the cell’s organelles, mitochondria and chloroplasts have their own DNA, and this DNA has the same form (a looped chromosome) as the DNA that’s found in bacteria.
- Both mitochondria and chloroplasts have their own ribosomes and produce some of their own proteins (though many mitochondrial and chloroplast genes have been transferred to the host cell, which also produces many mitochondrial and chloroplast proteins).
- Both mitochondria and chloroplasts replicate themselves autonomously (independently of the host cell’s cell cycle).
All of this evidence points to the same conclusion: that mitochondria and chloroplasts are themselves cells. They just happen to be cells that live inside of other cells.
And how do we know that the original host was an archaean? This idea is mostly based on molecular genetic similarities between eukaryotes and Archaeans. In both domains, the cells
- Package up their DNA by wrapping it around proteins called histones.
- Replicate their DNA using similar mechanisms.
4. Two Models for the Evolution of Endosymbiosis and Compartmentalization
The evidence listed above shows that both mitochondria and chloroplasts arose by endosymbiosis. How did this endosymbiosis happen? And did endosymbiosis precede compartmentalization, or result from it?
4a.Model 1: A compartmentalized proto-eukaryote engulfed a bacterium
In many textbooks (Campbell, Biology by Pearson; Principles of Life by Sinauer), the story of endosymbiosis and the origins of eukaryotic compartmentalization runs as follows.
Step 1: A prokaryotic cell (1) lost its cell wall, leaving it with a membrane (b) and genetic material (a).
Step 2: Infoldings of the membrane (not shown) caused the cell (shown at 2) to develop an internal membrane system, with an endoplasmic reticulum (d) and vacuoles (e).
Step 3: Without a wall, this cell (3) could now adopt a phagocytic lifestyle, engulfing other cells (such as the one at “f”)
Step 4: At some point, one engulfed bacterial cell (at “f”) was not digested for food, but managed to stay alive inside its host. This engulfed bacterium developed into an ATP-secreting mitochondrion (at “g”). This type of cell (at 4) became the ancestor of all eukaryotes.
Step 5: Later, a eukaryotic cell (one already possessing mitochondria, such as the one shown at “5”), engulfed a cyanobacterium (at “h”). Cyanobacteria are bacterial cells that perform photosynthesis.
Step 6: This engulfed cell evolved, over time, into the chloroplasts (at “j”) found in plants and algae.
To summarize: the basic idea behind this scenario is that eukaryotic compartmentalization evolved first (steps 1 and 2 above), and then endosymbiosis took place (steps 3 and 5).
4b. Model 2: Endosymbiosis led to compartmentalization
An alternative model is that endosymbiosis occurred first, and compartmentalization followed.
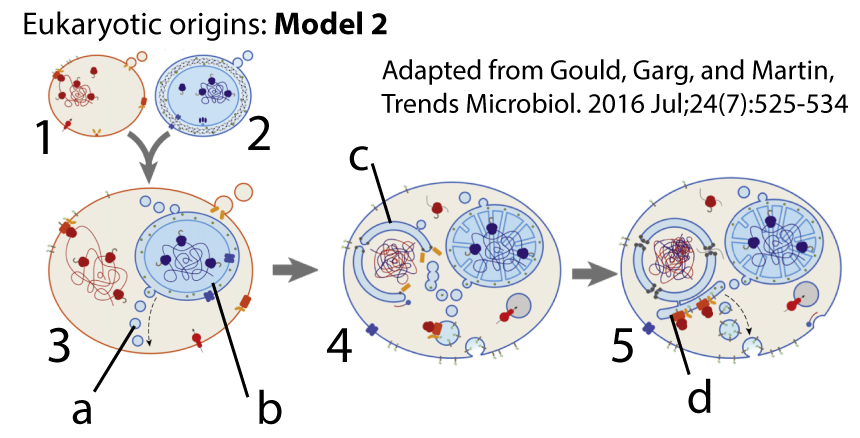
In this model, a bacterial cell (shown at 2) entered into an archaeal cell (at 1). Once inside, the bacterial cell (now a proto-mitochondrion, at “b”) secreted vesicles (shown at “a”) which accumulated inside its new host (cell “3”). In cell 4, these secreted vesicles become the source for the nuclear membrane (at “c”) and later, in cell 5, for the endoplasmic reticulum (shown at “d”) and, by extension, the other organelles of the endomembrane system.
Most textbooks, when explaining the origin of compartmentalization and the origin of eukaryotes, present a version of Model 1. Model 2, presented by biologists like William Martin and popularized by biochemist Nick Lane, seems to me to be the better bet. I suggest you learn both (and both diagrams are in the quiz below).
5. Checking Understanding
Next time you step on a scale, take that number and divide it by 10. That number is your mitochondrial mass: 10% of your body weight. By the numbers, you possess about 10 million billion (1 x 1015) mitochondria (Morgridge Institute). In a way, we animals are huge bacterial colonies: a mitochondrion’s way of making more mitochondria.
Let’s see how well you’ve mastered this material.
[qwiz qrecord_id=”sciencemusicvideosMeister1961-compartmentalization and endosymbiosis”] [h]
Cellular Compartmentalization: Checking Understanding
[i]Mitochondria
Making ATP
Setting the stage for
Life’s complexity
[q] Organelles like the mitochondria, the Golgi, and lysosomes are all examples of cellular [hangman].
[c]IGNvbXBhcnRtZW50YWxpemF0aW9u[Qq]
[f]IENvcnJlY3Qh[Qq]
[q] The only domain in which extensive cellular compartmentalization is found is domain [hangman].
[c]ZXVrYXJ5YQ==[Qq]
[f]IEV4Y2VsbGVudCE=[Qq]
[q] In the diagram below, which arrow shows the acquisition of a bacterial cell by an archaeal cell that led to mitochondria.
[textentry single_char=”true”]
[c]ID Q=[Qq]
[f]IEV4Y2VsbGVudC4gJiM4MjIwOzQmIzgyMjE7IHNob3dzIHRoZSBhY3F1aXNpdGlvbiBvZiBhIGJhY3RlcmlhbCBjZWxsIHRoYXQsIG92ZXIgdGltZSwgZXZvbHZlZCBpbnRvIHRoZSBtaXRvY2hvbmRyaWEu[Qq]
[c]IEVudGVyIHdvcmQ=[Qq]
[c]ICo=[Qq]
[f]IE5vLiBIZXJlJiM4MjE3O3MgYSBoaW50LiBNaXRvY2hvbmRyaWEgYXJlIGZvdW5kIGluIGFsbCBldWthcnlvdGljIGNlbGxzLiBXaGljaCBhcnJvdyBpcyBwb2ludGluZyB0byB0aGUgYmFzZSBvZiB0aGUgZXVrYXJ5b3RpYyBicmFuY2ggb2YgdGhlIHRyZWUgb2YgbGlmZT8=[Qq]
[q] In the diagram below, which arrow shows the acquisition of a bacterial cell by a eukaryotic cell that led to chloroplasts.
[textentry single_char=”true”]
[c]ID U=[Qq]
[f]IE5pY2UuICYjODIyMDs1JiM4MjIxOyBzaG93cyB0aGUgYWNxdWlzaXRpb24gb2YgYSBiYWN0ZXJpYWwgY2VsbCB0aGF0LCBvdmVyIHRpbWUsIGV2b2x2ZWQgaW50byB0aGUgY2hsb3JvcGxhc3Qu[Qq]
[c]IEVudGVyIHdvcmQ=[Qq]
[c]ICo=[Qq]
[f]IE5vLiBIZXJlJiM4MjE3O3MgYSBoaW50LiBDaGxvcm9wbGFzdHMgYXJlIGZvdW5kIGluIHBsYW50IGNlbGxzLiBXaGljaCBhcnJvdyBpcyBwb2ludGluZyB0byB0aGUgYmFzZSBvZiB0aGUgcGxhbnQga2luZ2RvbT8=[Qq]
[q]The [hangman] are a prokaryotic domain that are — based on their metabolism and molecular structure —actually quite different from bacteria.
[c]QXJjaGFlYQ==[Qq]
[q]Cells that lack a nucleus or other membrane-bound compartments are described as [hangman]
[c]cHJva2FyeW90aWM=[Qq]
[q]The cell type found in animals, plants, fungi, and many single-celled organisms with large complex cells is described as [hangman].
[c]ZXVrYXJ5b3RpYw==[Qq]
[q]The only domain that includes multicellular organisms is the [hangman].
[c]ZXVrYXJ5YQ==[Qq]
[q]A relationship in which one organism lives inside one another is called [hangman].
[c]ZW5kb3N5bWJpb3Npcw==[Qq]
[q]A symbiotic relationship in which both organisms benefit is called [hangman].
[c]bXV0dWFsaXNt[Qq]
[q]Mitochondria can be thought of as [hangman] endosymbionts living within every [hangman] cell. The mitochondrial supply their hosts with [hangman]. In return, the mitochondria receive [hangman] and oxygen.
[c]bXV0dWFsaXN0aWM=[Qq]
[c]ZXVrYXJ5b3RpYw==[Qq]
[c]QVRQ[Qq]
[c]Zm9vZA==[Qq]
[q]In most theories about the origin of mitochondria, the cell that took up the mitochondria would have belonged to the domain [hangman].
[c]YXJjaGFlYQ==[Qq]
[q]Evidence for the endosymbiotic origins of mitochondria and chloroplasts includes the fact that both mitochondria and chloroplasts have a [hangman] membrane; that both possess their own [hangman], which is organized into a looped [hangman]; and that both can [hangman] by binary fission, just like bacteria.
[c]ZG91Ymxl[Qq]
[c]RE5B[Qq]
[c]Y2hyb21vc29tZQ==[Qq]
[c]cmVwcm9kdWNl[Qq]
[q] In the simplified phylogenetic tree shown below, which number indicates the Domain Bacteria?
[textentry single_char=”true”]
[c]ID E=[Qq]
[f]IEV4Y2VsbGVudC4gJiM4MjIwOzEmIzgyMjE7IGluZGljYXRlcyB0aGUgYmFjdGVyaWEu[Qq]
[c]IEVudGVyIHdvcmQ=[Qq]
[c]ICo=[Qq]
[f]IE5vLiBIZXJlJiM4MjE3O3MgYSBoaW50LiBUaGUgZXVrYXJ5b3RlcyBhbmQgYXJjaGFlYSBhcmUgdGhlIHR3byBtb3N0IGNsb3NlbHkgcmVsYXRlZCBkb21haW5zLiBJZiBldWthcnlvdGVzIGFyZSBhdCAmIzgyMjA7MiwmIzgyMjE7IHdoZXJlIHdvdWxkIHRoZSBiYWN0ZXJpYSBoYXZlIHRvIGJlPw==[Qq]
[q] In the simplified phylogenetic tree shown below, which number or letter indicates mitochondria?
[textentry single_char=”true”]
[c]IG E=[Qq]
[f]IEV4Y2VsbGVudC4gJiM4MjIwO0EmIzgyMjE7IGluZGljYXRlcyB0aGUgbWl0b2Nob25kcmlhLg==[Qq]
[c]IEVudGVyIHdvcmQ=[Qq]
[c]ICo=[Qq]
[f]IE5vLiBIZXJlJiM4MjE3O3MgYSBoaW50LiBNaXRvY2hvbmRyaWEgYXJlIHRoZSBldm9sdXRpb25hcnkgaW5ub3ZhdGlvbiB0aGF0IGxlZCB0byB0aGUgZXVrYXJ5b3Rlcy4=[Qq]
[q] In the simplified phylogenetic tree shown below, which number or letter indicates the Domain Archaea?
[textentry single_char=”true”]
[c]ID M=[Qq]
[f]IEV4Y2VsbGVudC4gJiM4MjIwOzMmIzgyMjE7IGluZGljYXRlcyB0aGUgQXJjaGFlYS4=[Qq]
[c]IEVudGVyIHdvcmQ=[Qq]
[c]ICo=[Qq]
[f]IE5vLiBIZXJlJiM4MjE3O3MgYSBoaW50LiBUaGUgZXVrYXJ5b3RlcyBhbmQgYXJjaGFlYSBhcmUgdGhlIHR3byBtb3N0IGNsb3NlbHkgcmVsYXRlZCBkb21haW5zLiBBbmltYWxzIGFyZSBldWthcnlvdGVzLiBZb3UgZmlndXJlIG91dCB0aGUgcmVzdC4=[Qq]
[q] In the simplified phylogenetic tree shown below, which number or letter indicates the evolution of chloroplasts?
[textentry single_char=”true”]
[c]IG I=[Qq]
[f]IEV4Y2VsbGVudC4gJiM4MjIwO0ImIzgyMjE7IGluZGljYXRlcyB0aGUgYWNxdWlzaXRpb24gb2YgY2hsb3JvcGxhc3RzLg==[Qq]
[c]IEVudGVyIHdvcmQ=[Qq]
[c]ICo=[Qq]
[f]IE5vLiBIZXJlJiM4MjE3O3MgYSBoaW50LiBUaGUgY2hsb3JvcGxhc3RzIGFyZSB0aGUga2V5IGZlYXR1cmUgdGhhdCBkZWZpbmVzIHRoZSBwbGFudCBraW5nZG9tLg==[Qq]
[q] In the simplified phylogenetic tree shown below, which number or letter indicates the only group with complex, compartmentalized cells?
[textentry single_char=”true”]
[c]ID I=[Qq]
[f]IEV4Y2VsbGVudC4gJiM4MjIwOzImIzgyMjE7IGluZGljYXRlcyB0aGUgZXVrYXJ5b3RlcywgdGhlIG9ubHkgZG9tYWluIHdpdGggY29tcGxleCwgY29tcGFydG1lbnRhbGl6ZWQgY2VsbHMu[Qq]
[c]IEVudGVyIHdvcmQ=[Qq]
[c]ICo=[Qq]
[f]IE5vLiBIZXJlJiM4MjE3O3MgYSBoaW50LiBUaGlzIGlzIHRoZSBvbmx5IGRvbWFpbiB0aGF0JiM4MjE3O3M=IG5vdA==IHByb2thcnlvdGljLg==[Qq]
[q] In the diagram below, which number or letter indicates a mitochondrion in a cell that was ancestral to fungi, animals, and some unicellular eukaryotes?
[textentry single_char=”true”]
[c]IG c=[Qq]
[f]IEV4Y2VsbGVudC4gJiM4MjIwO0cmIzgyMjE7IGluZGljYXRlcyB0aGUgbWl0b2Nob25kcmlvbiBpbiBhIG5vbi1waG90b3N5bnRoZXRpYyBjZWxsLiBUaGlzIGNlbGwgd291bGQgaGF2ZSBiZWVuIHRoZSBhbmNlc3RvciB0byBmdW5naSwgYW5pbWFscywgYW5kIHRoZSBub24tcGhvdG9zeW50aGV0aWMgdW5pY2VsbHVsYXIgZXVrYXJ5b3RlcyAobGlrZSBwYXJhbWVjaWEgb3IgYW1vZWJhcyk=[Qq]
[c]IEVudGVyIHdvcmQ=[Qq]
[c]ICo=[Qq]
[f]IE5vLiBIZXJlJiM4MjE3O3MgYSBoaW50LiBGaW5kIGFuIGVuZG9zeW1iaW90aWMgb3JnYW5lbGxlIHRoYXQmIzgyMTc7cyBpbiBBTEwgZXVrYXJ5b3RlcywgYnV0IGluIGEgY2VsbCB0aGF0IGRvZXNuJiM4MjE3O3QgaGF2ZSBjaGxvcm9wbGFzdHMu[Qq]
[q] In the diagram below, the free-living bacterial cell that evolved into a mitochondrion is shown at
[textentry single_char=”true”]
[c]IG Y=[Qq]
[f]IEV4Y2VsbGVudC4gJiM4MjIwO0YmIzgyMjE7IGluZGljYXRlcyB0aGUgZnJlZS1saXZpbmcgYmFjdGVyaWFsIGNlbGwgdGhhdCBldm9sdmVkIHRvIGJlY29tZSBhIG1pdG9jaG9uZHJpb24u[Qq]
[c]IEVudGVyIHdvcmQ=[Qq]
[c]ICo=[Qq]
[f]IE5vLiBIZXJlJiM4MjE3O3MgYSBoaW50LiBUaGUgbWl0b2Nob25kcmlvbiBpcyB0aGUgZmlyc3Qgb3JnYW5lbGxlIHRvIGhhdmUgYXJpc2VuIGVuZG9zeW1iaW90aWNhbGx5[Qq]
[q] In the diagram below, the free-living photosynthetic bacterial cell that evolved into a chloroplast is shown at
[textentry single_char=”true”]
[c]IG g=[Qq]
[f]IEV4Y2VsbGVudC4gJiM4MjIwO0gmIzgyMjE7IGluZGljYXRlcyB0aGUgZnJlZS1saXZpbmcgcGhvdG9zeW50aGV0aWMgYmFjdGVyaWFsIGNlbGwgdGhhdCBldm9sdmVkIHRvIGJlY29tZSBhIGNobG9yb3BsYXN0Lg==[Qq]
[c]IEVudGVyIHdvcmQ=[Qq]
[c]ICo=[Qq]
[f]IE5vLiBIZXJlJiM4MjE3O3MgYSBoaW50LiBUaGUgY2hsb3JvcGxhc3QgaXMgdGhlIHNlY29uZCBvcmdhbmVsbGUgdG8gaGF2ZSBhcmlzZW4gdGhyb3VnaCBlbmRvc3ltYmlvc2lzLg==[Qq]
[q] In the diagram below, the endoplasmic reticulum is shown at
[textentry single_char=”true”]
[c]IG Q=[Qq]
[f]IEV4Y2VsbGVudC4gJiM4MjIwO0QmIzgyMjE7IGluZGljYXRlcyB0aGUgZW5kb3BsYXNtaWMgcmV0aWN1bHVt[Qq]
[c]IEVudGVyIHdvcmQ=[Qq]
[c]ICo=[Qq]
[f]IE5vLiBIZXJlJiM4MjE3O3MgYSBoaW50LiBUaGUgZW5kb3BsYXNtaWMgcmV0aWN1bHVtIGlzIGFuIGludGVybmFsIG5ldHdvcmsgb2YgY2hhbm5lbHMsIHN1cnJvdW5kZWQgYnkgbWVtYnJhbmUu[Qq]
[q]In the model of eukaryotic origins shown below, [hangman] (such as that which produced the ER and vacuoles) precedes [hangman].
[c]Y29tcGFydG1lbnRhbGl6YXRpb24=[Qq]
[c]ZW5kb3N5bWJpb3Npcw==[Qq]
[q] In the diagram below, an archaeal cell is shown at
[textentry single_char=”true”]
[c]ID E=[Qq]
[f]IE5pY2Ugam9iLiAmIzgyMjA7MSYjODIyMTsgaW5kaWNhdGVzIHRoZSBhcmNoYWVhbCBjZWxsIHRoYXQgdG9vayB1cCBhIGJhY3RlcmlhbCBjZWxsLCB0aGUgY2VsbHVsYXIgYWNxdWlzaXRpb24gdGhhdCBsZWQgdG8gdGhlIGV1a2FyeW90ZXMu[Qq]
[c]IEVudGVyIHdvcmQ=[Qq]
[c]ICo=[Qq]
[f]IE5vLiBIZXJlJiM4MjE3O3MgYSBoaW50LiBUaGUgYXJjaGFlYWwgY2VsbCB3YXMgdGhlIG9uZSB0aGF0LCBpbiB0aGUgYmVnaW5uaW5nIG9mIHRoZSBldWthcnlvdGljIHN0b3J5LCB0b29rIHVwIGEgYmFjdGVyaWFsIGNlbGwu[Qq]
[q] In the diagram below, vesicles secreted by the primitive mitochondria are shown at
[textentry single_char=”true”]
[c]IG E=[Qq]
[f]QXdlc29tZS4gVGhlIGxldHRlciAmIzgyMjA7YSYjODIyMTsgaW5kaWNhdGVzIHZlc2ljbGVzIGJlaW5nIHJlbGVhc2VkIGJ5IHRoZSBwcmltaXRpdmUgbWl0b2Nob25kcmlhLiBVbHRpbWF0ZWx5LCB0aGVzZSB2ZXNpY2xlcyB3aWxsIHByb3ZpZGUgdGhlIG1lbWJyYW5lIHRoYXQgd2lsbCBmb3JtIHRoZSBudWNsZWFyIG1lbWJyYW5lLCB0aGUgb3JnYW5lbGxlcyBvZiB0aGUgZW5kb21lbWJyYW5lIHN5c3RlbSwgYW5kIHRoZQ==[Qq]
[c]IEVudGVyIHdvcmQ=[Qq]
[c]ICo=[Qq]
[f]IE5vLiBIZXJlJiM4MjE3O3MgYSBoaW50LiBWZXNpY2xlcyBhcmUgZmx1aWQtZmlsbGVkIGJ1YmJsZXMgc3Vycm91bmRlZCBieSBhIG1lbWJyYW5lLg==[Qq]
[q] In the diagram below, the developing nuclear membrane is shown by which number or letter?
[textentry single_char=”true”]
[c]IG M=[Qq]
[f]VGVycmlmaWMuIExldHRlciAmIzgyMjA7QyYjODIyMTsgaW5kaWNhdGVzIHRoZSBkZXZlbG9waW5nIG51Y2xlYXIgbWVtYnJhbmUu[Qq]
[c]IEVudGVyIHdvcmQ=[Qq]
[c]ICo=[Qq]
[f]IE5vLiBIZXJlJiM4MjE3O3MgYSBoaW50LiBUaGUgbnVjbGVhciBtZW1icmFuZSBkZXZlbG9wcyBhcm91bmQgdGhlIGdlbmV0aWMgbWF0ZXJpYWwgdGhhdCB3YXMgb3JpZ2luYWxseSBpbiB0aGUgQXJjaGFlYWwgaG9zdCBjZWxsLg==[Qq]
[q] In the diagram below, the developing endoplasmic reticulum is shown by which number or letter?
[textentry single_char=”true”]
[c]IG Q=[Qq]
[f]VGVycmlmaWMuIExldHRlciAmIzgyMjA7ZCYjODIyMTsgaW5kaWNhdGVzIHRoZSBkZXZlbG9waW5nIGVuZG9wbGFzbWljIHJldGljdWx1bS4=[Qq]
[c]IEVudGVyIHdvcmQ=[Qq]
[c]ICo=[Qq]
[f]IE5vLiBIZXJlJiM4MjE3O3MgYSBoaW50LiBUaGUgRVIgaXMgc2hvd24gY2xvc2UgdG8gdGhlIG51Y2xlYXIgbWVtYnJhbmUu[Qq]
[q]In the model of eukaryotic origins shown below, [hangman] occurred first. The engulfed cell released [hangman] that developed into the nuclear [hangman], the endoplasmic [hangman], and the other organelles of the endomembrane system.
[c]ZW5kb3N5bWJpb3Npcw==[Qq]
[c]dmVzaWNsZXM=[Qq]
[c]bWVtYnJhbmU=[Qq]
[c]cmV0aWN1bHVt[Qq]
[x][restart]
[/qwiz]
Links
- Continue on to Cell Membranes (the next topic in this and most other curricula)
- Return to the Cell Structure and Function Main Menu
Further Reading
Both of the links below open in new tabs. The first is an article written for the general public by one of today’s best science writers, Carl Zimmer. The second is a peer-reviewed article by some of the best thinkers in the origin-of-life field.
- On the Origin of the Eukaryotes (Carl Zimmer)
- Bacterial Vesicle Secretion and the Evolutionary Origin of the Eukaryotic Endomembrane System
Notes:
We know that all life can be traced back to a single common ancestor because of the common features shared by all living things. These include use of DNA as genetic material, use of ATP for cellular energy, generation of ATP through a process called chemiosmosis, protein synthesis using the same genetic code, and so on. These shared features can be explained in only one of two ways: they were inherited from a common ancestor, and as a result are present in all of that ancestor’s descendants. Alternatively, they evolved independently over and over again, in every branch of the tree of life. Because the second alternative is unlikely, we reject it. All life is related. Every living thing is cousin to every other living thing.