1. Nepotism and Altruism in Belding’s Ground Squirrels
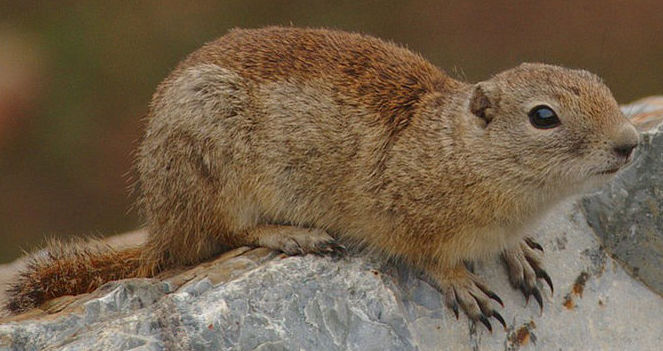
The Belding’s ground squirrel ranges from California’s Sierra Nevada mountains into Northeast Oregon; Southwest Idaho; and North and Central Nevada. Within this range, they prefer open, vegetated areas, where they feed on flowers, seeds, insects, and whatever else they can find. Within their habitat, they dig burrows where they spend the night. During the day, they forage for food.
The squirrels themselves are prey for a variety of carnivorous mammals (such as coyotes, bobcats, and weasels) as well as predatory birds (eagles and hawks). In response to threats from terrestrial predators, ground squirrels emit alarm calls: a series of five rapid notes. Squirrels that hear the alarm call will stand up on their back legs to look around, run on a rock to get a better view or return to their core area (Wikipedia). A different call is emitted in response to an aerial threat from a hawk or eagle.
One interpretation of these calls is that they’re examples of altruism: self-sacrificing behavior that reduces one individual’s fitness while increasing the fitness of others in the population. But altruistic behavior can be difficult to reconcile with Darwinian natural selection. Read this excerpt from the Stanford Encyclopedia of Philosophy.
Natural selection leads us to expect animals to behave in ways that increase their own chances of survival and reproduction, not those of others…By behaving altruistically an animal reduces its own fitness, so should be at a selective disadvantage vis-à-vis one which behaves selfishly.
To see this, imagine that some members of a group of Vervet monkeys give alarm calls when they see predators, but others do not. Other things being equal, the latter will have an advantage. By selfishly refusing to give an alarm call, a monkey can reduce the chance that it will be attacked, while at the same time benefiting from the alarm calls of others. So we should expect natural selection to favor those monkeys that do not give alarm calls over those that do.
To see if ground squirrel predator alarm calls were altruistic, Paul W. Sherman carried out a 3-year field study of predator alarm calls in Belding’s ground squirrels (see Science magazine, 23 September 1977).
Sherman’s study involved 3000 hours of observation over three summers. Sherman and his assistants marked the squirrels with ear tags and human hair dye for visual identification. Burrows were marked with sticks and painted rocks. Throughout the study, Sherman’s team observed 102 incidents of predatory activity by weasels, badgers, dogs (unaccompanied by humans), coyotes, and pine martens. Those 102 incidents resulted in 9 kills: six of adults and 3 of juveniles.
Here’s a piece of information for understanding the squirrel’s behavior.
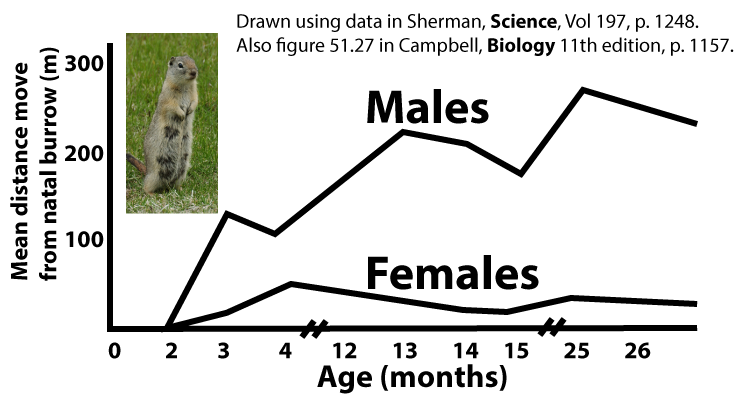
- In any small area within a ground squirrel colony, relatedness among females is high. This is because females are sedentary after breeding, and their daughters stay close to where they were born. For example, Sherman noted that 12 females born in 1974 mated within 43 meters of the burrows where they were born.
- Males emigrate from where they were born, seeking out new mates relatively far from their natal burrow. For example, Sherman noted that the seven males born in 1974, when they became sexually active in 1976, mated an average of 422 meters away from their natal burrow.
Use what you’ve read above (along with any other prior knowledge you might have about male and female behavior in mammals) to label the graph below.
[qwiz qrecord_id=”sciencemusicvideosMeister1961-Alarm calls in male and female ground squirrels (v2.0)”]
[h]Alarm calls in male and female ground squirrels
[q labels = “top”]The graph below shows the representation of adult males and females in the ground squirrel population (white bars on the left) and their observed frequency of giving alarm calls. Label each row as being emitted by a male or a female.
[l]male
[fx] No, that’s not correct. Please try again.
[f*] Good!
[l]female
[fx] No. Please try again.
[f*] Good!
[q]Let’s take a closer look at what Sherman observed. First of all, at any age, [hangman] are far more likely to give alarm calls than [hangman].
[c]ZmVtYWxlcw==[Qq]
[c]bWFsZXM=[Qq]
[q labels = “top”]Why are _________ so much more likely to give an alarm call than ________? Remember that the females _______ from the burrows where they were ________ much less than the males do. Because of that, any female is much more likely to live close to members of her immediate and extended __________. So, alarm calls aren’t exactly altruistic, because when a female risks herself to give an alarm, her behavior tends to help her ___________.
[l]born
[fx] No, that’s not correct. Please try again.
[f*] Correct!
[l]family
[fx] No. Please try again.
[f*] Excellent!
[l]females
[fx] No. Please try again.
[f*] Excellent!
[l]males
[fx] No. Please try again.
[f*] Great!
[l]move
[fx] No. Please try again.
[f*] Correct!
[l]relatives
[fx] No. Please try again.
[f*] Correct!
[q]A three-letter word for relative is [hangman] (hint: it begins with “k”). And the entire phenomenon at work with the ground squirrels can be thought of as an extension of natural [hangman] that’s called [hangman] selection (use the same word that you used earlier).
[c]a2lu[Qq]
[c]c2VsZWN0aW9u[Qq]
[c]a2lu[Qq]
[/qwiz]
2. Inclusive Fitness can Motivate Altruistic Behaviors
The title of Sherman’s published study is Nepotism and the Evolution of Alarm Calls. In human affairs, especially in democratic societies that are supposed to be meritocratic, nepotism has a pejorative feel to it. But among animals, nepotism reflects two evolutionary phenomena — inclusive fitness and kin selection — that can drive altruistic behaviors.
Here’s how this works: what persists in the population, over time, are genes: a body is only a temporary vessel for those genes. So if an allele programs an individual’s behavior so that they are willing to sacrifice their lives to save the lives of their offspring, it makes evolutionary sense. The sacrifice will have the effect of maintaining (or increasing, depending on the number of offspring saved) one’s genetic representation in the population.
By the same logic, it might also make sense to sacrifice oneself for other relatives, even if they’re not direct offspring. For example, I share half of my genes with my siblings. An allele that programmed my behavior to sacrifice myself for two siblings would be a 1:1 trade in terms of that allele’s representation in the gene pool. An allele that programmed me to sacrifice myself for 3 siblings would increase that allele’s representation in the gene pool.
Now extend that logic. My cousins share not 1/2, but 1/8 of my genes. So, it would make sense for me to sacrifice myself to preserve the lives of 9 cousins.
Note the nuance here. The older I am, the higher the chance that I’ll no longer directly pass on my genes, and the higher the chance that I have already passed on these genes to my offspring. Therefore, the older I am, the more it makes sense for me to sacrifice myself to maintain those genes within the bodies of my relatives. On the other hand, the older my siblings or cousins are, the less sense it makes for me to sacrifice myself for them.
This is the idea behind inclusive fitness. Fitness isn’t about just an individual organism’s reproductive success: it’s also about the survival and successful reproduction of those genes in related individuals.
A mathematical model for inclusive fitness was developed by William Hamilton in 1964. In Hamilton’s model, there are three key variables:
- B is the benefit to the recipient of the altruistic behavior, measured by the average number of extra offspring that the recipient will produce.
- C is the cost to the altruist, measured by how many fewer offspring the altruist will produce.
- r is the coefficient of relatedness, measured by the fraction of genes that the altruist and recipient share.
Natural selection will favor altruism when
rB>C
an equation that has come to be known as Hamilton’s rule.
In plain English, it means that if the recipients are closely related to me (r), and my behavior results in more offspring for them (B), then that outweighs the cost (C) of my own altruistic behavior.
Let’s see this in action.
A mother squirrel sees a hawk overhead, above her daughter. If the daughter is eaten by the hawk, it loses all chance of producing offspring. If it survived, it might have produced two offspring throughout its life (assuming that the population of squirrels is a stable one that replaces itself). So, the benefit to the daughter is two offspring (B = 2). Now, assume that the risk to the mother, by calling out is 25% (she has a 25% chance of dying because the hawk’s attention is drawn to her). Mom is getting on in years, and she might have one more pup, so her cost is 0.25 x 1 = 0.25). The mother shares half of her genes with her daughter, so the coefficient of relatedness, r, is 0.5. Plugging in all of these values, we have
rB = 0.5 x 2 = 1.0
C = 0.25 x 1 = 0.25
So, in this case, because rB > C, then we can expect the mother to engage in altruistic behavior, despite the risk.
As stated above, because inclusive fitness involves sacrificing oneself for relatives, it’s often referred to as Kin Selection.
Now, this seems well and fine for humans. After all, I know who my children, cousins, and siblings are. But how do animals know who their kin are? One possibility involves recognition mechanisms. For example, the Belding ground squirrel produces both oral and anal secretions, and kin produce similar odors. Squirrels, in experimental settings, spend more time investigating non-kin scents than kin scents (indicating that they can tell the difference)
Another possibility is much simpler, and also applies to ground squirrels. If you’re a female, altruistic alleles program you to behave altruistically because those individuals around you are likely to be your kin. The same altruistic alleles, in a male body, don’t produce the same behavior.
3. Nepotism, Inclusive Fitness, and Altruism: Checking Understanding
Note that this discussion of altruism and inclusive fitness only scratches the surface of a very deep (and relatively young) field. Another process resulting in altruistic behavior is reciprocal altruism, which you can read about on Wikipedia, or, here (if you’re up for reading an article by Robert Trivers, one of the original thinkers in this field. Or you can read the book that made me become a biology teacher: The Selfish Gene, by Richard Dawkins (the link goes to a Wikipedia article).
[qwiz qrecord_id=”sciencemusicvideosMeister1961-Nepotism, Inclusive Fitness, and Altruism (v2.0)”]
[h]Nepotism, Inclusive Fitness, and Altruism
[i]Biohaiku
Inclusive fitness:
Altruistic Behavior
Comes from selfish genes
[q] Self-sacrificing behavior that reduces one organism’s fitness while increasing the fitness of others in the population is called[hangman].
[c]IGFsdHJ1aXNt[Qq]
[q]Sherman’s study of alarm calls in ground squirrels found (among other things) that [hangman] squirrels were much less likely to emit alarm calls than were [hangman]. That’s because the calls are examples of [hangman] (begins with an “n”): behavior that benefits close relatives.
[c]bWFsZQ==[Qq]
[c]ZmVtYWxlcw==[Qq]
[c]bmVwb3Rpc20=[Qq]
[q]Nepotism can be explained through [hangman] selection, a phenomenon that is closely related to the idea of [hangman] fitness.
[c]a2lu[Qq]
[c]aW5jbHVzaXZl[Qq]
[q]The idea of kin selection works like this. I share approximately [hangman] of my genes with each of my siblings and an [hangman] of my genes with each of my cousins. Therefore, behavior in which I risk myself to save [hangman] siblings or [hangman] cousins will increase the frequency of my genes in my gene [hangman ].
[c]aGFsZg==[Qq]
[c]ZWlnaHRo[Qq]
[c]dGhyZWU=[Qq]
[c]bmluZQ==[Qq]
[c]cG9vbA==[Qq]
[q]The concept of inclusive fitness has been modeled by the equation rB>C, also known as Hamilton’s Rule. In this expression, “r” is the coefficient of [hangman]. “C” is the [hangman] of the behavior to the altruist, and “B” is the [hangman] the altruistic behavior will have for its recipients.
[c]cmVsYXRlZG5lc3M=[Qq]
[c]Y29zdA==[Qq]
[c]YmVuZWZpdA==[Qq]
[q]There are two ways for inclusive fitness to work. 1) Relatives must live in [hangman] proximity to one another. In that scenario, helping those around me helps my [hangman], increasing the representation of my [hangman] in the gene pool. Alternatively, there must be a mechanism by which I can recognize my [hangman], allowing me to direct my altruistic behavior towards them. In both cases, altruism is really [hangman].
[c]Y2xvc2U=[Qq]
[c]cmVsYXRpdmVz[Qq]
[c]Z2VuZXM=[Qq]
[c]a2lu[Qq]
[c]bmVwb3Rpc20=[Qq]
[x][restart]
[/qwiz]
4. Inclusive Fitness, Eusociality, and Haplodiploidy
Inclusive fitness goes a long way toward explaining the evolution of what’s called eusociality. Eusociality is a social structure in which some individuals within a colony breed, while others are non-reproductive. Think of a beehive. A beehive has one reproducing queen and tens of thousands of sterile workers who gather food and care for the young. Among the insects, eusociality is found in bees, ants, wasps (which are all in the order Hymenoptera), and termites. It’s also found in one species of shrimp and two species of mole rats.
Eusociality involves a lot of altruistic behavior: the worker bees forego their own reproduction and instead work for the good of the hive. The workers’ lives are spent raising the queen’s offspring, and sometimes workers give their lives to defend the hive (since delivering a sting kills the stinging bee).
In what follows, we’re going to focus on one of several factors that explain eusociality: a genetic structure called haplodiploidy. In haplodiploid species, females are diploid, and develop from fertilized eggs, while males are haploid, developing from unfertilized eggs. Take a moment to study the diagram below.
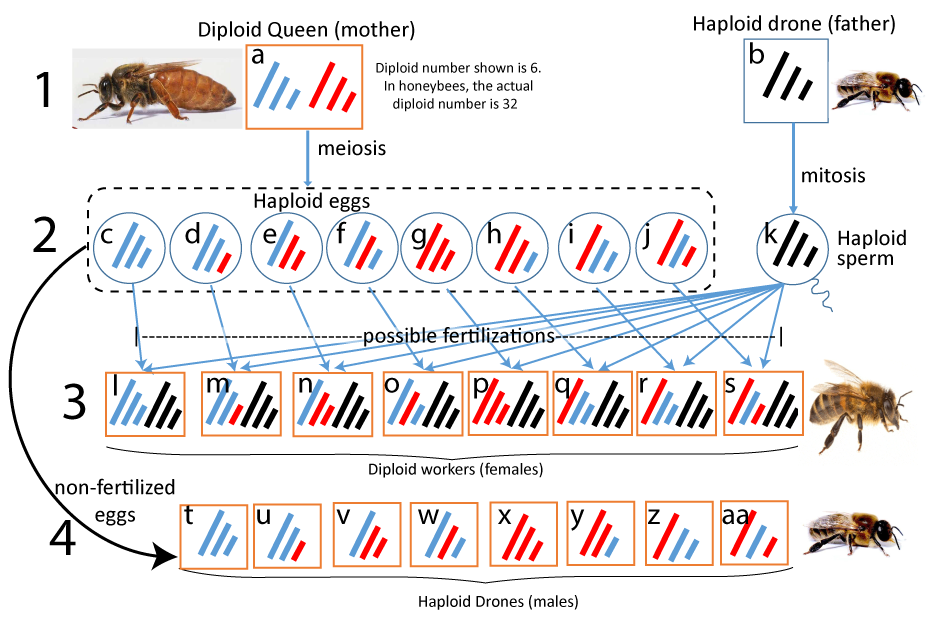
From a genetic perspective, there are only three types of individuals in a beehive.
- Queens are female, diploid, and capable of reproducing.
- Workers are female, diploid, but (almost always) sterile. All of the workers are sisters (daughters of the queen)
- Drones are male and haploid. Unlike the worker bees, they neither gather pollen nor defend the hive. Their only job is to mate with an unfertilized queen.
Because the drones are haploid, they can’t perform meiosis. Rather, when a drone produces sperm, he passes on 100% of his genome through his haploid sperm.
The queen, by contrast, can perform meiosis. As with any set of haploid eggs produced by a diploid female, the queen’s fertilized eggs will have 50% of her chromosomes, with the other 50% coming from the father. Queens also lay unfertilized eggs which develop into haploid drones. Note that a small proportion of worker bees also lay unfertilized eggs, but not to an extent undermines that basic idea below.
In the diploid zygotes that develop into female workers, there’s one set of chromosomes from the father and a second set from the mother. Because of haplodiploidy and the father’s haploid status, all of the paternal chromosomes (and the alleles they carry) are 100% identical in every worker in the hive. The second set of chromosomes that a worker bee inherits from her mother (the queen) has a 50% similarity. Average together the 100% genetic similarity from their father with the 50% similarity from their mother, and we get an average of 75% relatedness between any one worker and any other.
You can get a sense of this by looking at row 3 in the diagram above. In the two sisters represented by “l” and “m,” 5 of the six chromosomes are identical, which means that these sisters share 83% of their genes. Sisters “l” and “n” are a bit less closely related: they share 66% of their genes (four of their six chromosomes). Note that because this diagram shows cells with a diploid number of six, the amount of relatedness between the sisters is underrepresented (because the highest amount of relatedness is 86%). With more chromosomes that number gets higher, causing the overall relatedness to average out at 75%. (see this article in Wikipedia)
In terms of inclusive fitness, haplodiploidy has some interesting consequences. For example, imagine that a worker was born who could mate and bear offspring. These offspring would have half of her genes (in the same way that a human mother shares half of her genes with her daughters or sons). That means that her own offspring would be less related to her than her worker bee sisters are (because all the sisters are, on average, 75% related to one another). Thus, in terms of maximizing the representation of one’s genes in the next generation, it makes more sense for a worker to assist her mother in creating more sisters than it does to reproduce on her own. And from this, we have a hive where everyone is working to support the queen. It’s all about inclusive fitness and kin selection.
The reality of haplodiploidy is more complex: for one thing, queens often mate with multiple drones, so the workers are not necessarily full sisters. Additionally, haplodiploidy, while it’s often associated with eusociality, doesn’t necessarily lead to eusociality (there are haplodiploid species that are not eusocial). Finally, some eusociality, such as the kind found in naked mole rats and termites, occurs in diploid organisms, with both males and females evolving from fertilized eggs.
5. Eusociality and Haplodiploidy: Checking Understanding
[qwiz qrecord_id=”sciencemusicvideosMeister1961-Eusociality and Haplodiploidy (v2.0)”]
[h]Eusociality and Haplodiploidy
[i]
[q] [hangman] is a social structure in which some individuals within a colony breed, while others are non-reproductive.
[c]IEV1c29jaWFsaXR5[Qq]
[f]IEV4Y2VsbGVudCE=[Qq]
[q] In[hangman] species, the females are diploid and develop from [hangman]eggs, while the males are [hangman] and develop from [hangman] eggs.
[c]IGhhcGxvZGlwbG9pZA==[Qq]
[f]IEV4Y2VsbGVudCE=[Qq]
[c]IGZlcnRpbGl6ZWQ=[Qq]
[f]IEdvb2Qh[Qq]
[c]IGhhcGxvaWQ=[Qq]
[f]IEdyZWF0IQ==[Qq]
[c]IHVuZmVydGlsaXplZA==[Qq]
[f]IEdvb2Qh[Qq]
[q] In terms of chromosome numbers, both the queen and the workers in a beehive are [hangman]. However, while the queen is fertile, the workers are [hangman]. The male drones, in terms of chromosome number, are [hangman].
[c]IGRpcGxvaWQ=[Qq]
[c]IHN0ZXJpbGU=[Qq]
[c]IGhhcGxvaWQ=[Qq]
[q] Because the drones are haploid, they can’t perform [hangman]. Their sperm cells contain 100% of their [hangman].
[c]IG1laW9zaXM=[Qq]
[c]IGdlbm9tZQ==[Qq]
[q]In haplodiploid species (such as bees), the workers share, on average [hangman]-five percent of their genes. By contrast, in species that aren’t haplodiploid, sisters share [hangman] percent of their genes.
[c]c2V2ZW50eQ==[Qq]
[c]ZmlmdHk=[Qq]
[q]The high degree of relatedness among the [hangman] in a bee colony goes a long way to explain the high degree of [hangman] behavior seen in a beehive. The best way to explain this is through the idea of [hangman] selection. That’s because a beehive is really one very close [hangman]. By working for the hive, the workers increase the representation of their own [hangman] in their gene pool.
[c]d29ya2Vycw==[Qq]
[c]YWx0cnVpc3RpYw==[Qq]
[c]a2lu[Qq]
[c]ZmFtaWx5[Qq]
[c]Z2VuZXM=[Qq]
[x]
[restart]
[/qwiz]
6. Honey Bee Dances
We saw above how haplodiploidy in bees leads to cooperation among the workers in the hive. One of the most important aspects of this cooperation involves foraging for nectar and pollen. Honeybee cooperation requires communication. When a honeybee worker finds a food source, how does it tell its fellow workers where it is?
The answer was determined by Karl von Frisch. Frisch made his discoveries in the 1940s and won the 1973 Nobel Prize for his work (along with Niko Tinbergen and Konrad Lorenz).
When a honeybee returns to its hive after successful foraging, it performs a dance that indicates the direction and distance of the source of its food.
The dance involves a figure-eight pattern. It begins with a waggle run (a), followed by a circular turn to the right (b), followed by another waggle run, followed by a circular turn to the left (c). The duration of the waggle run is proportional to the distance between the hive (bottom left) and the food source (top right). The intensity of the waggle reflects how excited the bee is about the resource.
The direction of the waggle run indicates the direction of the resources. If the bee runs straight up, then the resource is directly in line with the sun (wherever the sun is in the sky relative to the hive).
In the example shown above and to the left, the workers would know that once they leave the hive and locate the sun, they should fly at an angle that’s about 30 degrees to the right of the sun to find the reported food.
Watch the video below.
Finally, while the discussion above has focused on communication within honeybees, note that the plants are also communicating. Through flower color, shape, and markings, plants are advertising a reward for pollinators (bees, butterflies, hummingbirds, etc.). That makes flower color a relatively small evolutionary investment on a plant’s part to forge a strong association with a particular pollinator. Color “teaches” pollinators to associate a particular flower color with the pollen or nectar that a pollinator can find by visiting that flower. Once this association is made, the pollinators will tend to focus more exclusively on flowers of that color, increasing the probability of pollen transfer between flowers of the same species.
7. Honey Bee Dances: Checking for Understanding
Note that some of the questions in the quiz below are from the video above (so make sure you watch the video first).
[qwiz qrecord_id=”sciencemusicvideosMeister1961-Honey bee dances (v2.0)”]
[h]Honey bee dances
[i]
[q] The duration of the waggle run performed by a bee that’s discovered a new food source is proportional to the [hangman] of its newly discovered food source.
[c]IGRpc3RhbmNl[Qq]
[q] If a bee performing a waggle dance does it in a straight down direction, then she’s telling her sisters to leave the hive, find the sun, and fly directly [hangman] from it to find the resource.
[c]IGF3YXk=[Qq]
[q] Because bees can perceive ultraviolet and [hangman]light, they can find the location of the sun at all times, even on the cloudiest days.
[c]IHBvbGFyaXplZA==[Qq]
[q] A circle dance (one that barely has a waggle portion) means that the food source i is very [hangman] to the hive.
[c]IGNsb3Nl[Qq]
[q]The duration of the waggle portion of the dance isn’t directly indicating distance, but rather the amount of [hangman] a bee needs to expend to get from the hive to the source of food.
[c]ZW5lcmd5[Qq]
[/qwiz]
What’s Next?
This tutorial ends AP Bio Topic 8.1 (Responses to the Environment/Animal Behavior). To continue with AP Bio Unit 8, please go to the AP Bio Unit 8 Main Menu.