Looking for a student learning guide? It’s linked in the main menu for your course. Use the “Courses” menu above.
1. Early Earth was Different from Present-Day Earth
Earth today has environments that would be difficult for you and me to survive in. Imagine, for example being dropped into the middle of the broiling Sahara desert or the frozen interiors of Greenland or Antarctica. But today’s Earth is a garden planet compared to how our planet was in its infancy.
In the previous tutorial, we learned that microfossils and fossilized stromatolites show clear evidence of life on Earth by 3.5 bya, with less convincing evidence for life even earlier than that. What was our planet like when life first emerged? Take a look at the depiction of early Earth below, and write down a few observations in your student learning guide.
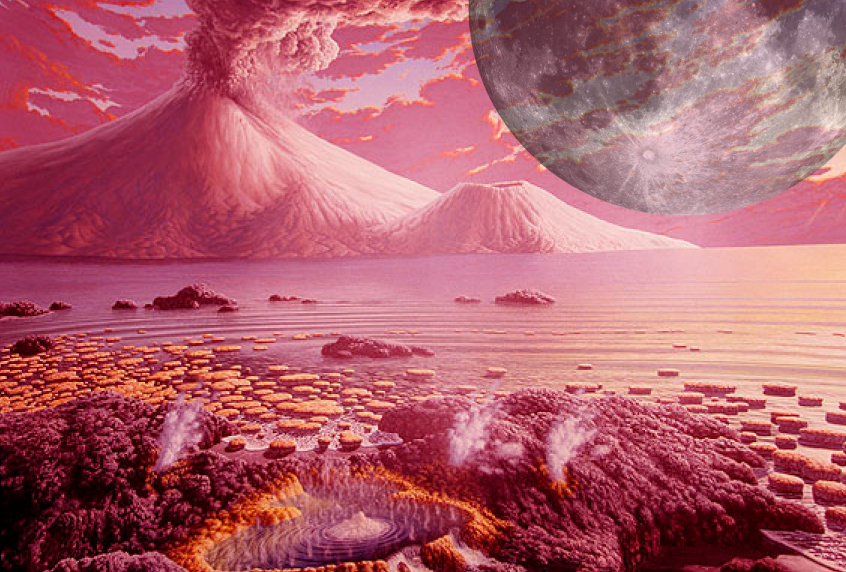
The period that spans the time from the Earth’s formation 4.6 bya until about 4 bya was so hostile that it’s been named the Hadean eon. The word “Hadean” is derived from Hades, the land of the dead in ancient Greek mythology, and is roughly synonymous with the word “hell.” Following the Hadean period is the Archaean eon, which spans the period from 4.0 to 2.5 bya. Sometime during the Hadean eon, or within one or two hundred million years of the start of the Archean eon, life emerged on planet Earth. Here’s what this hellish world was like.
1a. The atmosphere lacked free oxygen (O2)
The red sky depicted above indicates the different compositions of the Hadean and Archaean atmospheres. In terms of the origin of life, the most important difference was the lack of atmospheric oxygen (O2). Today, our atmosphere is about 21% oxygen. That oxygen is there because plants and photosynthetic bacteria have, for the past several billion years, been splitting water molecules apart and releasing molecular oxygen. Before life emerged, there would have been no photosynthesis and no free oxygen.
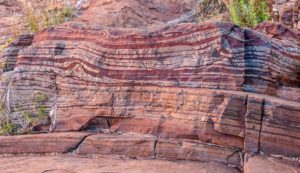
There are multiple lines of evidence for early Earth’s oxygen-free atmosphere. Minerals from the early Earth include iron-based compounds that could not have formed in the presence of oxygen. That’s because oxygen is an oxidizing agent that steals electrons from other compounds. When elemental iron is exposed to oxygen, the iron is oxidized to rust. Huge deposits of rust (such as the banded iron formation shown at right) start to form on Earth at about 2.4 bya, a period that is associated with the first accumulation of photosynthetically originated oxygen in the seas.
The atmospheres of the other rocky planets (Venus and Mars) are also models for what Earth’s atmosphere would be like in the absence of life. Take a moment and study the table below:
Mercury | Venus | Earth | Mars | |
CO2 | 0.0% | 96.5% | 0.03% | 95.3% |
N2 | 0.0% | 3.5% | 78% | 2.7% |
Ar | 0.0% | 0.007% | 1% | 1.6% |
O2 | 0.0% | 0.0% | 21% | 0.13% |
H2O | 0.0% | 0.002% | 0.1% | 0-0.1% |
The key point is the complete lack (on Venus) or trace amount (on Mars) of molecular oxygen in the atmospheres of the other planets of the solar system.
So what gases were in early Earth’s atmosphere? Probably mostly nitrogen gas (N2) and carbon dioxide (CO2). These gases were either emitted from volcanoes or arrived in the comets and meteors that were bombarding the Earth at that time.
1b. Meteor and Comet Strikes were Common
The entire Hadean and the early Archaean eon is referred to as the period of heavy bombardment. Earth, during this time, was being pummeled by meteors and comets. These impacts would have released enormous amounts of energy, melting the crust in the surrounding area.
1c. The Moon was Much Closer
The moon is thought to have formed as a result of a massive impact between Earth and another planet that was about a third of the Earth’s size (approximately the size of Mars). The moon formed from the debris that was flung into space. When the moon first coalesced, it was 10 to 20 times closer (source: space.com) than it is now. Tidal forces exerted by the Moon would have been much stronger than now (because gravitational force varies as the square of the distance between two objects, so reducing the distance by ten times would have increased the gravitational force by 100 times).
1d. There was more volcanic activity
Volcanic activity is caused by heat within the Earth. Most of that heat comes from radioactive decay. A younger Earth would have had more radioactive isotopes, more heat, and more volcanic activity.
1e. There was very little land
The continents have grown over time through a process called accretion. Today, the Earth’s surface is about 30% land and 70% ocean. On early Earth, the amount of land would have been less. Once the Earth had cooled enough to allow for liquid water to accumulate on the surface, that surface would have been covered by a global ocean, with a few volcanic islands peeking through (source: New Scientist).
2. Early Earth: Checking Understanding
Some of the conditions described above would have made the emergence of life impossible on the surface of our young planet impossible. These include meteor impacts that would have melted the crust and destroyed any early emerging life forms. Other conditions, such as the lack of oxygen in the atmosphere, were conducive to the emergence of life. That’s because oxygen, with its propensity for stealing electrons, can destroy complex molecules like nucleic acids and proteins. A lack of oxygen thus might have promoted the emergence of life on Earth. As we’ll see in what follows, other features of the young Earth give us clues about where and how life might have arisen. But first, let’s consolidate your learning.
[qwiz random = “true” qrecord_id=”sciencemusicvideosMeister1961-Early Earth, Checking Understanding”]
[h]Conditions on the Early Earth
[i]
[q] Unlike our current atmosphere, the early Earth’s atmosphere was almost certainly lacking in [hangman]
[c]IG94eWdlbg==[Qq]
[f]IEdyZWF0IQ==[Qq]
[q] During the period of heavy [hangman], there were frequent impacts of meteors and [hangman].
[c]IGJvbWJhcmRtZW50[Qq]
[f]IEdvb2Qh[Qq]
[c]IGNvbWV0cw==[Qq]
[f]IEV4Y2VsbGVudCE=[Qq]
[q] If you were able to visit the Earth about 3.5 bya, the days would be shorter. In the evening, you’d see a much brighter and closer [hangman]. As a result, you’d have to be careful if you approached the shore because there would be much higher [hangman]. And, you’d have to remember to bring some kind of breathing apparatus, because of the lack of [hangman] in the air.
[c]IE1vb24=[Qq]
[f]IEV4Y2VsbGVudCE=[Qq]
[c]IHRpZGVz[Qq]
[f]IENvcnJlY3Qh[Qq]
[c]IG94eWdlbg==[Qq]
[f]IENvcnJlY3Qh[Qq]
[q] In the young Earth, there would be much more activity from [hangman]. A few of these would poke above the surface of a world-covering [hangman], creating the only land surfaces.
[c]IHZvbGNhbm9lcw==[Qq]
[f]IEdyZWF0IQ==[Qq]
[c]IG9jZWFu[Qq]
[f]IEdyZWF0IQ==[Qq]
[q multiple_choice=”true”] Which of the following dates is most likely for the origin of life?
[c]IDQuNiBieWE=[Qq]
[f]IE5vLiBUaGF0JiM4MjE3O3Mgd2F5IHRvbyBlYXJseS4gVGhhdCYjODIxNztzIHdoZW4gdGhlIEVhcnRoIHdhcyBmaXJzdCBmb3JtaW5nLg==[Qq]
[c]IDQuMSBieWE=[Qq]
[f]IE5vLiBUaGF0JiM4MjE3O3MgZHVyaW5nIHRoZSBwZXJpb2Qgb2YgaGVhdnkgYm9tYmFyZG1lbnQuIEFueSBlbWVyZ2luZyBsaWZlIHByb2JhYmx5IHdvdWxkIGhhdmUgYmVlbiBkZXN0cm95ZWQgYnkgaW5jb21pbmcgbWV0ZW9ycyBhbmQgY29tZXRzLg==[Qq]
[c]IDMuOC BieWE=[Qq]
[f]RXhjZWxsZW50ISBZb3UmIzgyMTc7dmUgY2hvc2VuIGEgZGF0ZSB0aGF0JiM4MjE3O3MgYmV0d2VlbiB0aGUgZW5kIG9mIHRoZSBwZXJpb2Qgb2YgaGVhdnkgYm9tYmFyZG1lbnQsIGFuZCBiZWZvcmUgdGhlIGRhdGUgZm9yIHRoZSBlYXJsaWVzdCBmb3NzaWxpemVkIGxpZmUuIExpZmUgaGFkIHRvIGVtZXJnZSBzb21ld2hlcmUgaW4gYmV0d2Vlbi4=[Qq]
[c]IDMuNSBieWE=[Qq]
[f]IE5vLiBBcyBzaG93biBieSBmb3NzaWxpemVkIHN0cm9tYXRvbGl0ZXMgYW5kIGFzc29jaWF0ZWQgbWljcm9mb3NzaWxzIGZyb20gdGhhdCBwZXJpb2QsIGxpZmUgd2FzIGFscmVhZHkgd2VsbCBlc3RhYmxpc2hlZCBieSAzLjUgYnlhLiBDaG9vc2UgYW4gZWFybGllciBwZXJpb2Qu[Qq]
[q] One piece of evidence for the oxygen-free atmosphere of early Earth is the presence of certain [hangman] containing minerals that could not have formed if oxygen were present in the air.
[c]IGlyb24=[Qq]
[q] The oxygen in our atmosphere is the product of [hangman], which splits water molecules apart.
[c]IHBob3Rvc3ludGhlc2lz[Qq]
[q] During photosynthesis, water is combined with carbon dioxide to produce carbohydrates. Oxygen is released as a waste product. The source of the oxygen is [hangman].
[c]IHdhdGVy[Qq]
[/qwiz]
3. Origin of Life: Key steps
In what follows, we’ll learn about how the first populations of living cells could have emerged from non-living matter; how chemistry developed into biology.
The table below shows some of the key steps required for the emergence of the first living cells. Use the biology you know (and logic) to fill in the blanks. Note that step 1 had to come first, but there’s no consensus on the sequence of the steps after that (and they could have all happened at the same time).
The one term you might not know is “abiotic.” It means “without life,” or “non-living.” For example, in ecology, we talk about abiotic factors such as air temperature or rainfall, while predatory animals are a biotic factor.
[qwiz qrecord_id=”sciencemusicvideosMeister1961-Key Steps in the Origin of Life: Interactive Table”]
[h]Interactive Table: Key Steps in the Origin of Life
[i]An origin of life haiku
When life first emerged
Was it heredity first,
Or metabolism?
[q labels= “right”]
FIRST | Abiotic creation of ___________ (the molecular building blocks of life) | |||
NEXT | Abiotically link monomers to form _________ | Origin of _____________. Create a self-perpetuating system for processing matter and _________; and for removing wastes. | Origin of _____________. Provide the system with a way to pass on ___________ for maintenance, growth, and reproduction | Encapsulate the system with a ____________ to keep it from dissolving away, creating the first primitive _______. |
[l]cells
[fx] No, that’s not correct. Please try again.
[f*] Correct!
[l]energy
[fx] No, that’s not correct. Please try again.
[f*] Correct!
[l]heredity
[fx] No. Please try again.
[f*] Excellent!
[l]instructions
[fx] No. Please try again.
[f*] Great!
[l]metabolism
[fx] No. Please try again.
[f*] Excellent!
[l]membrane
[fx] No. Please try again.
[f*] Great!
[l]monomers
[fx] No, that’s not correct. Please try again.
[f*] Great!
[l]polymers
[fx] No, that’s not correct. Please try again.
[f*] Good!
[q labels= “top”]Here’s the same table, but with different blanks to fill in.
FIRST | __________ creation of monomers (the molecular__________ blocks of life. | |||
NEXT | Abiotically link ___________ to form polymers | Origin of metabolism. Create a self-____________ system for processing _______ and energy and for removing _______ | Origin of heredity. Provide the system with a way to ________ instructions about maintenance, growth, and _____________. | ___________ the system with a membrane to keep it from dissolving away, creating the first primitive cells. |
[l]Abiotic
[fx] No, that’s not correct. Please try again.
[f*] Good!
[l]building
[fx] No, that’s not correct. Please try again.
[f*] Correct!
[l]Encapsulate
[fx] No, that’s not correct. Please try again.
[f*] Correct!
[l]matter
[fx] No, that’s not correct. Please try again.
[f*] Correct!
[l]monomers
[fx] No. Please try again.
[f*] Great!
[l]pass on
[fx] No, that’s not correct. Please try again.
[f*] Great!
[l]perpetuating
[fx] No. Please try again.
[f*] Great!
[l]reproduction
[fx] No. Please try again.
[f*] Great!
[l]wastes
[fx] No. Please try again.
[f*] Correct!
[q]So, what’s the probability that all of this (or any of this) could happen on its own? That’s the key to understanding the origin of life. How could something as complex as life arise from much less complex non-life? Let’s take it step by step.
[/qwiz]
4. Abiotic Formation of Monomers: Oparin, Haldane, and The Miller-Urey Experiment
Let’s address the first step in the table you completed above: the abiotic creation of monomers.
NASA’s working definition of life defines it as a “self-sustaining chemical system capable of Darwinian evolution.” All living things on Earth are built of four types of molecules: nucleic acids, proteins, lipids, and carbohydrates. Each class of molecule (lipids excepted) is a type of polymer, composed of simpler monomers. So, to explain the origin of the first cells, we have to be able to explain the origin of monomers.
All of these monomers have a few traits in common.
- They’re small (compared to polymers), but they can contain up to a dozen atoms or more. Study the table below, which shows a few common monomers
Note that “small” and “simple” are relative terms. Monomers are small and simple compared to the proteins and nucleic acids in modern cells, which can have thousands or even millions of atoms. Compared to molecules like water (3 atoms) and carbon dioxide (3 atoms), these monomers are big.
- They’re all chemically reduced, high-energy molecules. They’re full of hydrogen atoms, which have highly energetic electrons. Three of the molecules shown are either used directly (ATP) or indirectly (glucose and caprylic acid) to power cellular work.
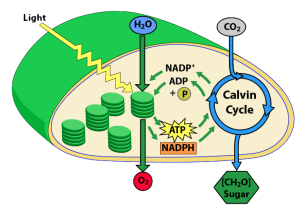
Today, monomers are made biotically. Plants and other autotrophs (self-feeding organisms) can make all of their monomers from oxidized, low-energy, simple molecules like carbon dioxide and water. Specifically, during photosynthesis plants combine carbon dioxide and water to make the three-carbon sugar G3P (glyceraldehyde 3 phosphate), releasing oxygen as a waste product. This reaction is endergonic: it has a positive ΔG and is powered by solar energy from the sun.
The molecular machinery that underlies photosynthesis is intricate and complex, and it’s an example of what life does: channeling energy and matter in such a way that creates a little current of order in a universe that’s always growing more disorderly. So, what we have to explain is how, in the absence of life, the reduced, high-energy substances that life is made of (the monomers and then the polymers) could come about.
In the 1920s, two scientists, Alexander Oparin and J.B.S. Haldane, independently hypothesized a way that this could happen. Under the conditions that were then thought to have prevailed on the early Earth, they thought that monomers could spontaneously arise. These monomers would accumulate in the early oceans, forming what’s called a primordial or prebiotic soup. This soup would subsequently serve as the source of polymers.
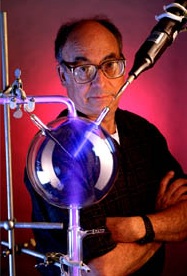
Oparin and Haldane’s thinking was informed by their thinking about the makeup of the early Earth’s atmosphere. They knew, as we know today, that early Earth’s atmosphere would have been oxygen-free (because they knew that molecular oxygen results from photosynthesis). They proposed, instead, that the early atmosphere would be chemically reducing. Reducing substances, as opposed to oxidizing substances like oxygen, contain high-energy electrons. Hydrogen gas and methane, for example, are both highly reduced gases. In chemical reactions, they lose electrons, and that loss releases energy. In the case of hydrogen, this type of electron donation can power a fuel cell to create electricity. In the case of methane, the electron donation can power combustion, either in an oven (creating heat) or in a gas-powered turbine (creating electricity).
In an oxidizing atmosphere, the spontaneous direction of chemical reactions is to break down complex molecules into simpler ones, like carbon dioxide and water. Think about what happens to metals in the modern atmosphere. Over time, they rust, which is a type of oxidation. Organic materials, like wood, burn (another type of oxidation). By contrast, Oparin and Haldane thought that in a reducing atmosphere, the opposite would occur. More complex molecules would form from simpler ones. And that, thought Oparin and Haldane is how the first monomers would arise.
In 1953, this idea, also known as the Oparin-Haldane hypothesis, was tested by Stanley Miller, who at that time was a 23-year-old graduate student at the University of Chicago. Miller was working with Harold Urey, a winner of the Nobel Prize for chemistry. Their experiment is now known as the Miller-Urey Experiment. For an atmospheric mix, Miller chose to use the gases that surround the outer, gas giant planets (like Jupiter and Saturn). These planets have highly reducing atmosphere that consists of methane (CH4), molecular hydrogen (H2), water, and ammonia (NH3).
The entire apparatus was a closed, sterile system, with various valves that could be used to vacuum out all air from the system (3), and sample what the system was producing without contaminating it (9). The system contained a water-filled chamber that simulated the ancient ocean (2). A heat source (1) boiled the ocean, creating water vapor that would rise into a chamber that represented the primitive atmosphere (6) with methane (CH4), ammonia (NH3), molecular hydrogen (H2), and water vapor (H2O)
The apparatus also contained electrodes (4) which could produce sparks (5), simulating lightning. A condenser (7) cooled the circulating gas. The cooled gas condensed as a liquid in a trap (8), which allowed Miller to see what his apparatus was brewing up.
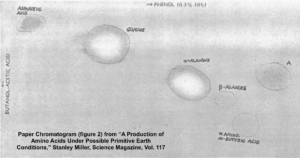
After five days, Miller stopped the experiment and sampled the contents. To detect what had formed within his apparatus, Miller used a technique called paper chromatography. This involves using solvents that rise up a paper column, carrying with them dissolved substances, which will rise a known amount in a specific period of time. You can see the results in the photograph Miller published in his May 1953 paper, which was entitled “A Production of Amino Acids under Possible Primitive Earth Conditions” (click the previous link to read: it’s only two pages). The specific amino acids Miller detected were aspartic acid, glycine, alpha and beta-alanine, and α-aminobutyric acid. Abiotic synthesis of monomers seemed to have been confirmed, and Miller’s experiment was widely reported in the press.
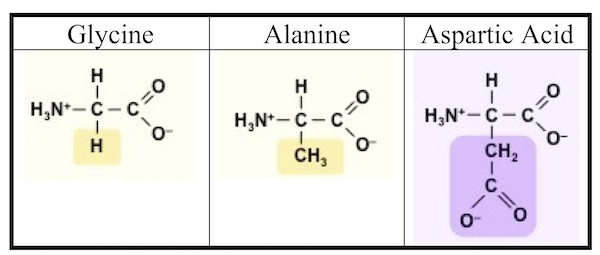
It turns out that Miller might have been more successful than even he knew. In 2007, the year Miller died, preserved results of his earliest experiments (his work from 1953, and in subsequent years) were reanalyzed. It was found that Miller’s spark-chamber experiments had yielded not five amino acids, but thirty-one, along with 12 dipeptides (two amino acids linked together by a peptide bond). Source: “One of the Foremost Experiments of the Twentieth Century.”
On the other hand, Miller’s work has been criticized for using an atmospheric mix that was too reducing, and thus too conducive to the formation of organic compounds. The Earth’s ancient atmosphere probably didn’t have significant amounts of molecular hydrogen or methane. But Miller’s work inspired many other experiments, which have used different mixes of gases, energy sources, and minerals (such as iron), and have resulted in the production of the nitrogenous bases found in RNA and DNA (though not complete nucleotides) and a wide array of other amino acids.
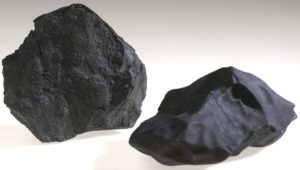
In addition, amino acids and nucleotide bases have been found inside meteorites (which form out in space) and have also been detected in comets. This includes the Murchison meteorite, which landed in Australia in 1969 and was subject to chemical analysis. What’s the takeaway? It seems probable that within a few hundred million years of the Earth’s cooling, some of the monomers that would make up the polymers within the first living organisms might have spontaneously formed.
We’ll look at scenarios for polymer formation in the next tutorial. But first, let’s consolidate our understanding of the Oparin-Haldane hypothesis and the Miller-Urey experiment.
5. The Miller Urey Experiment: Checking Understanding
[qwiz random = “true” qrecord_id=”sciencemusicvideosMeister1961-Miller-Urey Experiment”]
[h]The Miller-Urey Experiment and Monomer Formation.
[i]
[q] In the Miller-Urey experiment, which number represents part that simulated the ancient oceans?
[textentry single_char=”true”]
[c]ID I=
[f]IEV4Y2VsbGVudC4gTnVtYmVyIDIgcmVwcmVzZW50cyB0aGUgd2F0ZXIgaW4gdGhlIGFuY2llbnQgb2NlYW5zLg==[Qq]
[c]ICo=[Qq]
[f]Tm8uIEhlcmUmIzgyMTc7cyBhIGhpbnQuIFdoaWNoIHBhcnQgbG9va3MgbGlrZSBpdCB3b3VsZCBjb250YWluIHdhdGVyPw==[Qq]
[q] In the Miller-Urey experiment, which number represents a part that simulated lightning in the ancient atmosphere?
[textentry single_char=”true”]
[c]ID U=
[f]IE5pY2Ugam9iLiBOdW1iZXIgNSByZXByZXNlbnRzIGxpZ2h0bmluZyBpbiB0aGUgYW5jaWVudCBhdG1vc3BoZXJlLg==[Qq]
[c]ICo=[Qq]
[f]Tm8uIEhlcmUmIzgyMTc7cyBhIGhpbnQuIFdoaWNoIHBhcnQgbG9va3MgbGlrZSBpdCB3b3VsZCByZXByZXNlbnQgYW4gZWxlY3RyaWNhbCBzcGFyaz8=[Qq]
[q] In the Miller-Urey experiment, which number represents a part that simulated heat from volcanoes?
[textentry single_char=”true”]
[c]ID E=
[f]IEdvb2Qgd29yay4gTnVtYmVyIDEgcmVwcmVzZW50cyBoZWF0IGZyb20gYW5jaWVudCB2b2xjYW5vZXMu[Qq]
[c]ICo=[Qq]
[f]Tm8uIEhlcmUmIzgyMTc7cyBhIGhpbnQuIFdoaWNoIHBhcnQgbG9va3MgbGlrZSBpdCB3b3VsZCBwcm9kdWNlIGhlYXQ/[Qq]
[q] In the diagram below of the Miller-Urey experiment, the electrodes that produce the lighting are represented by which number?
[textentry single_char=”true”]
[c]ID Q=
[f]V2F5IHRvIGdvLiBOdW1iZXIgNCByZXByZXNlbnRzIHRoZSBzcGFyay1wcm9kdWNpbmcgZWxlY3Ryb2Rlcy4=[Qq]
[c]ICo=[Qq]
[f]Tm8uIEhlcmUmIzgyMTc7cyBhIGhpbnQuIEVsZWN0cmljaXR5IGhhcyBwb3NpdGl2ZSBhbmQgbmVnYXRpdmUgY2hhcmdlcy4gV2hlcmUgZG8geW91IHNlZSBzeW1ib2xzIHRoYXQgd291bGQgcmVwcmVzZW50IHBsdXMgYW5kIG1pbnVzIGNoYXJnZXM/[Qq]
[q] In the diagram below of the Miller-Urey experiment, the chamber that contains the gases in the ancient atmosphere would be found at which number?
[textentry single_char=”true”]
[c]Ng ==
[f]Q29ycmVjdC4gTnVtYmVyIDYgcmVwcmVzZW50cyB0aGUgYW5jaWVudCBhdG1vc3BoZXJlLg==[Qq]
[c]ICo=[Qq]
[f]Tm8uIEhlcmUmIzgyMTc7cyBhIGhpbnQuIFRoZSBsaWdodGluZyBvY2N1cnMgd2l0aGluIHRoZSBhdG1vc3BoZXJlLg==[Qq]
[q] In the diagram below of the Miller-Urey experiment, the condenser that cooled the gases in the atmosphere, causing whatever was in the atmosphere to precipitate into the trap, is found at which number?
[textentry single_char=”true”]
[c]Nw ==
[f]TmljZS4gTnVtYmVyIDcgcmVwcmVzZW50cyB0aGUgY29uZGVuc2VyLg==[Qq]
[c]ICo=[Qq]
[f]Tm8uIEhlcmUmIzgyMTc7cyBhIGhpbnQuIENvbmRlbnNlcnMgY2lyY3VsYXRlIHdhdGVyIHRoYXQgY2F1c2VzIHZhcG9ycyB0byBjb25kZW5zZS4gRmluZCB3aGVyZSB3YXRlciBpcyBjaXJjdWxhdGluZyBpbiBhbmQgb3V0Lg==[Qq]
[q] In the diagram below of the Miller-Urey experiment, where would amino acids and other organic compounds have been collected?
[textentry single_char=”true”]
[c]OA ==
[f]TmljZS4gTnVtYmVyIDggcmVwcmVzZW50cyB0aGUgdHJhcCwgYW5kIHRoYXQmIzgyMTc7cyB3aGVyZSBvcmdhbmljIGNvbXBvdW5kcyBsaWtlIGFtaW5vIGFjaWRzIHdvdWxkIHByZWNpcGl0YXRlIGZvciBjb2xsZWN0aW9uLg==[Qq]
[c]ICo=[Qq]
[f]Tm8uIEhlcmUmIzgyMTc7cyBhIGhpbnQuIFRoZSB0cmFwIGlzIGJlbmVhdGggdGhlIGNvbmRlbnNlci4=[Qq]
[q]In the Miller-Urey experiment, the mixture of gases used in the experiment differed from the gases in the current atmosphere in many ways. For one thing, [hangman] (a gas produced by photosynthesis) was lacking.
[c]b3h5Z2Vu[Qq]
[q]Because of the presence of oxygen, today’s atmosphere is oxidizing. In the Oparin-Haldane hypothesis, the atmosphere is thought to be [hangman].
[c]cmVkdWNpbmc=[Qq]
[q]The Miller-Urey experiment was designed to prove that [hangman] synthesis of monomers might have been possible on the early Earth
[c]YWJpb3RpYw==[Qq]
[q]According to the Oparin-Haldane hypothesis, the abiotic synthesis of monomers would result in the creation of a [hangman] soup, in which life would subsequently emerge.
[c]cHJpbW9yZGlhbA==[Qq]
[x][restart]
[/qwiz]